Wellness may finally be having its day as a critical function of building design.
Over the last decade, architects and engineers have been working to educate owners and developers on the wellness impacts of sustainable building design approaches in creating healthier and more productive lives for their occupants. However, the COVID-19 pandemic has demonstrated that these wellness considerations are no longer a mere value-add to a building, but rather they are a necessary element in allowing larger groups to safely reoccupy a space. Before we can go back to work, each building owner and operations team is going to have to consider what wellness integrations need to be put in place before tenants can return.
Buildings already designed to LEED, WELL, or Fitwel requirements have a leg up in this area. Designing for environmental health and lower carbon impacts have long been key selling points of these rating systems, but each feature criteria that can mitigate the spread of COVID-19 and help occupants adjust safely to a new normal.
While it’s important to note that none of the criteria we review here can wholly prevent the spread of the virus, these strategies, coupled with social distancing, can help make spaces safer.
Ventilation Effectiveness
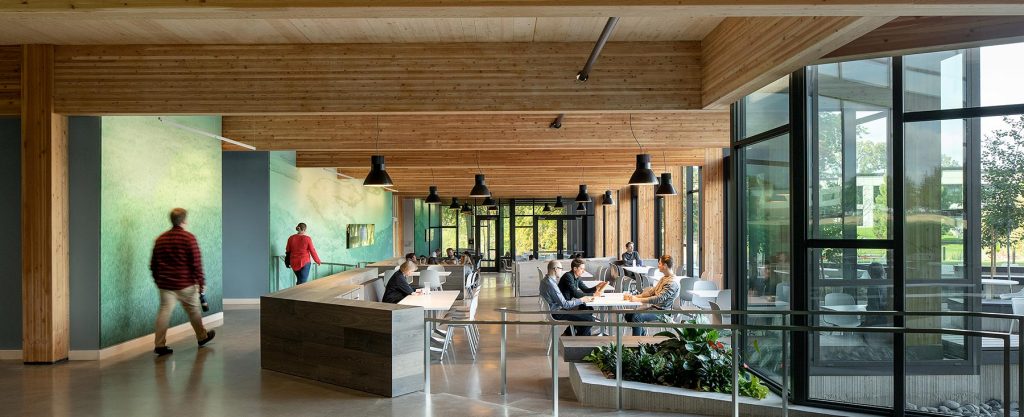
One easy way to limit the build-up of viruses and bacteria within indoor air is to get rid of them! Mechanical air handling systems are meant to supply fresh oxygenated air to indoor spaces and exhaust carbon dioxide, particulate matter, formaldehyde, carbon monoxide, and a host of harmful compounds like VOC’s from the air. In the process, virus particles are exhausted too, but if the building’s ventilation and air change rates are insufficient, a higher concentration of virus particles may remain in the breathing space. It is well known that COVID-19 is spread when an infected person sneezes or coughs expelling droplets containing millions of little microbes. They can become aerosolized in the breathing space or land on touchable surfaces.
WELL Air Precondition 3 and LEED v4.1 O+M (Indoor Environmental Quality [EQ] Prerequisite 1) require ventilation systems to be tested and balanced every five years and maintained to ASHRAE 62.1 air supply rates. This best practice should be adopted by building operators to ready their buildings for reopening as air supply rates can, and often do, drop below adequate levels as changes are made to system operations, building space use, and as equipment ages.
With well-designed ventilation systems and periodic maintenance, building operators can minimize the spread of the virus. More so, increasing air supply by 30% over ASHRAE 62.1 (recommended by WELL Air Optimization 6 and LEED v4.1 BD+C EQ Credit 1) serves to provide additional fresh air that can help dilute the concentration of the virus in the breathing space. A higher air supply rate is standard practice in the healthcare industry and given the risk of COVID-19, it’s a good time to adopt this practice and tune/retrofit existing building equipment to bring in additional fresh air. Sensors added to the air supply systems can tie to the Building Management System providing regular air supply and air quality monitoring (WELL Air Optimization 8).
To receive Sustainability Matters and articles like this in your inbox, Sign Up Here
It is also great practice to open windows and doors, if at all possible, to increase air flow through buildings. WELL and LEED promote operable windows (WELL Air Optimization 7; LEED v4.1 BD+C EQ Prerequisite 1) and openings.
All of these air quality measures can be documented in an Indoor Air Quality Policy for the building and Fitwel Indoor Environment strategy 6.3 provides framework for its development.
Beyond good engineering and maintenance practices to reduce the virus’s spread, a secondary benefit that may ease the discomfort and concerns around returning to work are integrating practices that improve occupant satisfaction. People sense they are in a healthy building when there is great daylight and the air smells fresh and not stagnant. The healthy feeling can be enhanced by open windows or doors and help occupants feel energized from sunlight and clear views to nature. The ability to easily access the outdoors via terraces and balconies further supports the health benefits. Occupants feel safer and more comfortable when their environment supports their health. By coupling good design and operations practice with connections to nature and biophilia (WELL Mind Precondition 2 and Fitwel Workspaces strategies 7.1-7.2), health conditions can be improved resulting in less stress returning to work.
Air Filtration
It’s common practice that outdoor air supply systems include some level of filtration to remove particulates, dust, and debris from entering the indoor environment. However, the level of filtration and filter maintenance is important to deliver fresh, healthy air to occupants. And, given the heightened concerns employees have for returning to the office, we can expect more questions and scrutiny of the air they’re breathing. That makes now a good time to establish filter replacement protocols that meet manufacturer recommendations and to evaluate whether the building’s air supply equipment can accommodate higher levels of filtration.
WELL Air Optimization 12 requires replacement records and establishes filter type by outdoor air conditions. Dense urban areas, hot and dry inland areas, as well as locations near transportation hubs and major highways likely have greater levels of particulate matter necessitating greater filtration. LEED (LEED v4.1 BD+C IEQ Credit 1) recommends a minimum MERV 13 filter and WELL Air Optimization 12 recommends up to MERV 16, with MERV 8 prefilters based on a review of outdoor air quality and particulate matter (called PM2.5 and PM10) levels. PM2.5 are the smallest air particulates that enter the lungs and blood stream causing heart disease and other cardiovascular complications.
MERV 13 is a superior filter and it can capture up to 75% of PM2.5 and bacteria and viruses and MERV 16 can capture up to 95% and could be of special consideration if the building recirculates indoor air. Above and beyond LEED and WELL credit, would be the use of MERV 17 or higher-grade filters classified as HEPA.
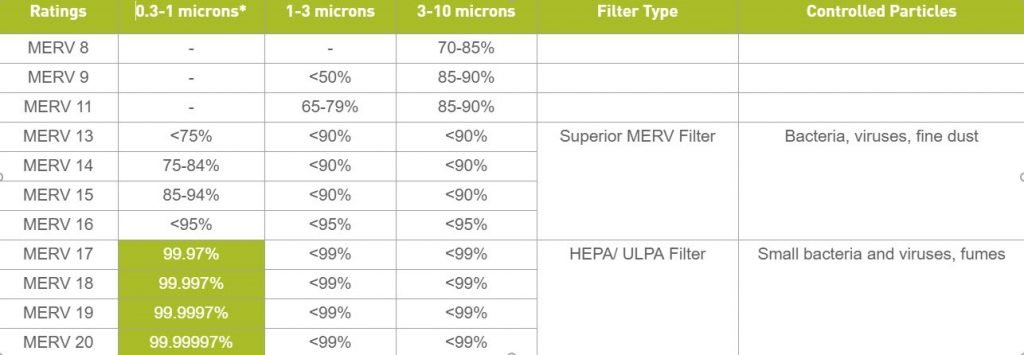
The COVID-19 virus is tiny – about 30 nanometers in diameter (for context, the diameter of an average hair follicle is about 80,000 nanometers). So small it alone will pass through most air filters on its own. However, it often catches a ride on particulate matter like PM2.5, coagulating into a piece of larger matter. Therefore, it is important that a minimum of MERV 13 filtration is used to filter out these particles, if not higher levels of filtration if the ventilation system can accommodate. These higher levels of filtration are powerful enough to mitigate (though, again, not prevent) the virus’s spread. For this reason, we are not recommending the installation of HEPA filters in commercial spaces. These filter types should be reserved for healthcare and critical facilities.
If systems cannot accommodate these larger filter sizes and associated pressure drop, electronic systems could be employed such as bipolar ionization to kill bacteria and viruses. WELL offers credit for UV treatment to treat mold and mildew spores on the cooling coils and drain pans of forced-air cooling systems (WELL Air Optimization 14). While this strategy works well in this instance, it will be ineffective in treating the virus because as it is traveling through the HVAC system, there is simply not enough residence time for the UV to do its work. The air simply passes through too quickly. Alternatively, standalone UV systems or UV lights can be used in spaces after hours to sterilize air and surfaces as part of a regular cleaning protocol.
LEED, WELL, and Fitwel also offer credit for testing indoor air quality. While standard ASHRAE 62.1 air quality test protocols don’t cover the detection of viruses, its implementation can help to demonstrate to occupants that the best practices you’ve implemented in the building are working and are thereby likely to protect against the spread of the virus (LEED v4.1 O+M EQ Prerequisite 4, WELL Air Precondition 1 and Optimization 5, and Fitwel Indoor Environment strategy 6.4).
Humidity Control
Certain humidity levels can enable viruses to survive longer and even grow in number making it harder to control their spread. High humidity may promote the accumulation and growth of microbes and low relative humidity has also been associated with longer survival (slower inactivation) rates.
“Recent data on indoor humidity and the spread of the virus recommends maintaining an RH range of between 40% and 60%”
The solution is for buildings in climates with broad humidity ranges to maintain relative humidity levels by adding or removing moisture from the air. The practice inhibits and improves air quality and thermal comfort. WELL Comfort Optimization 7 recommends that the mechanical systems in all projects (except spaces designed for high humidity such as natatoriums and greenhouses), have the ability to maintain relative humidity (RH) between 30% and 60% at all times. The modeled RH levels in the space must also remain within this range for at least 98% of all business hours of the year. Recent data on indoor humidity and the spread of the virus recommends maintaining an RH range of between 40% and 60%. The recommended range serves to maintain larger droplets that contain viral particles, thus causing them to deposit onto room surfaces more quickly instead of remaining aerosolized in the breathing space. It is also thought that there is higher likelihood for the membranes to be disrupted and inactivated. And below this range, low ambient humidity hurts the ability of the immune system to fight respiratory viral infections.
Cleaning Products and Protocol
This is a great time to evaluate building janitorial cleaning practices.
LEED offers credit for green cleaning practices (LEED v4.1 O+M EQ Prerequisite 3 and EQ Credit 6-7), but WELL Materials Optimization 9 and Fitwel Shared Spaces strategies 8.1-8.2 and 8.4 go beyond the types of cleaning products used and offer additional rigor for training and protocols that could be especially helpful in mitigating the spread of the COVID-19 virus.
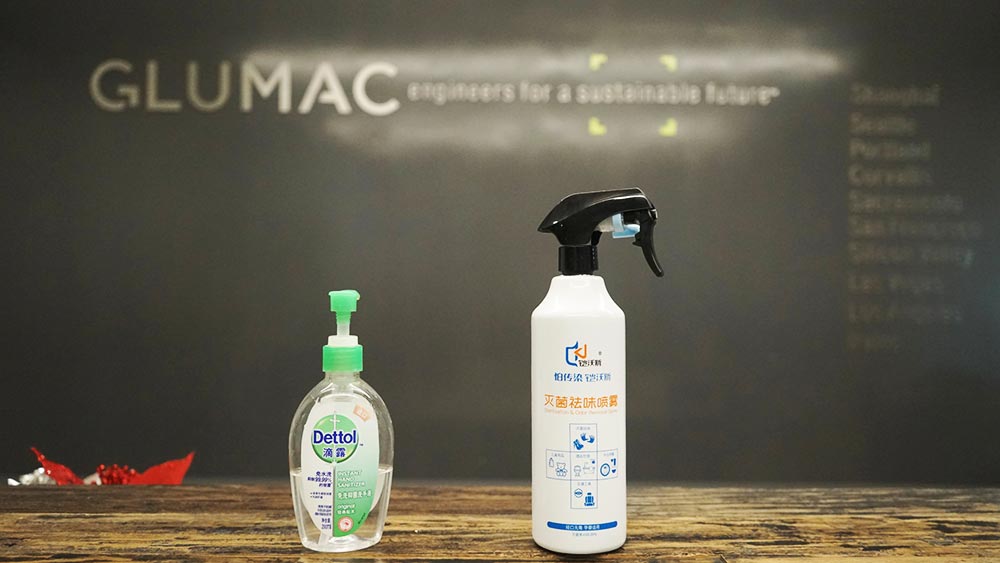
The WELL guidance could help building operations teams to develop more comprehensive cleaning programs. WELL covers training on the sequence of cleaning steps and the use of personal protective equipment. And the standard covers cleaning protocols, specifically the extent and frequency of cleaning including dated cleaning logs as well as protocols for disinfection, including the identification and maintenance of a list of high-touch surfaces and their limitations to disinfection. WELL Water Optimization 8 further supports cleanliness by offering building occupant guidance for proper hand-washing, specifically for sink and faucet design, soap dispensers, and hand drying.
In comparison, Fitwel delineates its guidance by higher risk locations, specifically protocols for bathroom cleaning (strategy 8.1) and break areas (strategy 8.4) and also provides signage guidance for proper hand washing (strategy 8.2).
Confidence in Your Strategy
There is no catch all approach to remove the risk of COVID-19 from your building. There are too many variables when moving people in an out of a space. What’s key to mitigating its spread – along with proper social distancing – is discovering which approaches will be most effective. We’re offering a comprehensive presentation that illustrates the variety of system upgrades, operational approaches, and hygienic techniques that can help create safer, healthier work environments. If you are interested in a digital presentation, please send an inquiry to [email protected].
UPDATES TO WELL AND LEED THE WEEK OF 5/28/20:
To help bring focus to strategies from the WELL Building Standard to support in the fight against COVID-19, The International WELL Building Institute (IWBI) just launched a PDF resource that brings focus to the strategies described here and more to include company risk management planning and mental and community resilience and recovery.
The US Green Building Council (USGBC) announced plans to release new LEED pilot credits for COVID-19 health and safety building strategies. Pilot credits can be swapped into your project scorecard for credit under the LEED Innovation in Design credit category. USGBC also announced upgrades to LEED v4.1 to prioritize health and a report or certificate for spaces well prepared for building re-entry.
Rendering Luman building in West LA courtesy of Gensler
Along with much of China, our Shanghai team has already begun returning to its office. It’s a high-performance space with some of the best air quality in the country. But during a pandemic, adjustments need to be made to maintain the health and safety of our staff.
Life is different. The basic ways we navigate our city and our office have changed in a lot of ways – big and small. The ways we get to work, how we eat, how we interact with each other and clients. That’s led to a new normal. Here’s what that new normal looks like, and how we’re keeping each other safe while working toward a sustainable future.
Commutes: Public Transportation
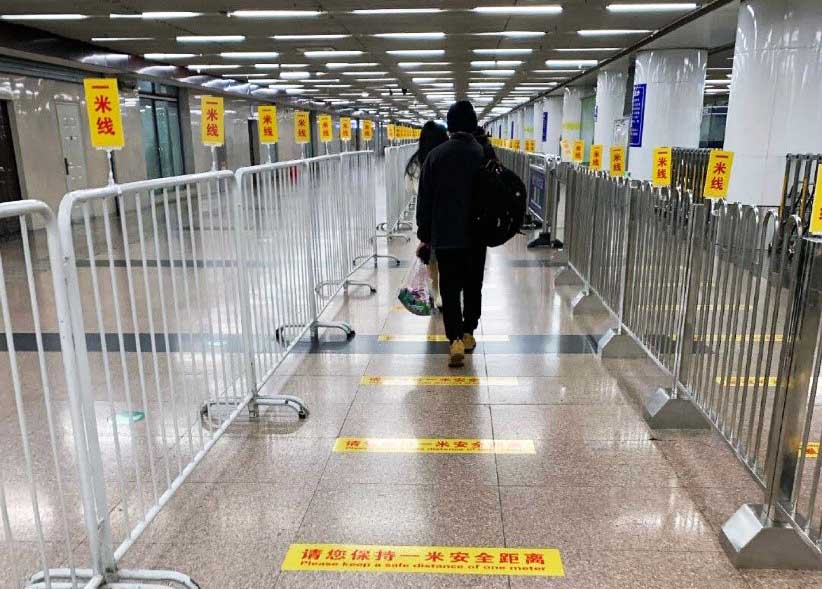
For many in Shanghai, commuting is a mix of public transportation and ride share. While both of these are still active, the experience has changed. The entrance to the subway is now marked off with guidelines for social distancing – following the recommendation from the World Health Organization, though it is slightly less than what is recommended by the United States Centers for Disease Control & Prevention. And riders are scanned an infrared radiation thermometer to test their temperature before entering the train. As fever is one of the COVID-19 virus’s key symptoms, this helps inform people that they may need to seek a COVID-19 test and if necessary begin quarantine and treatment.
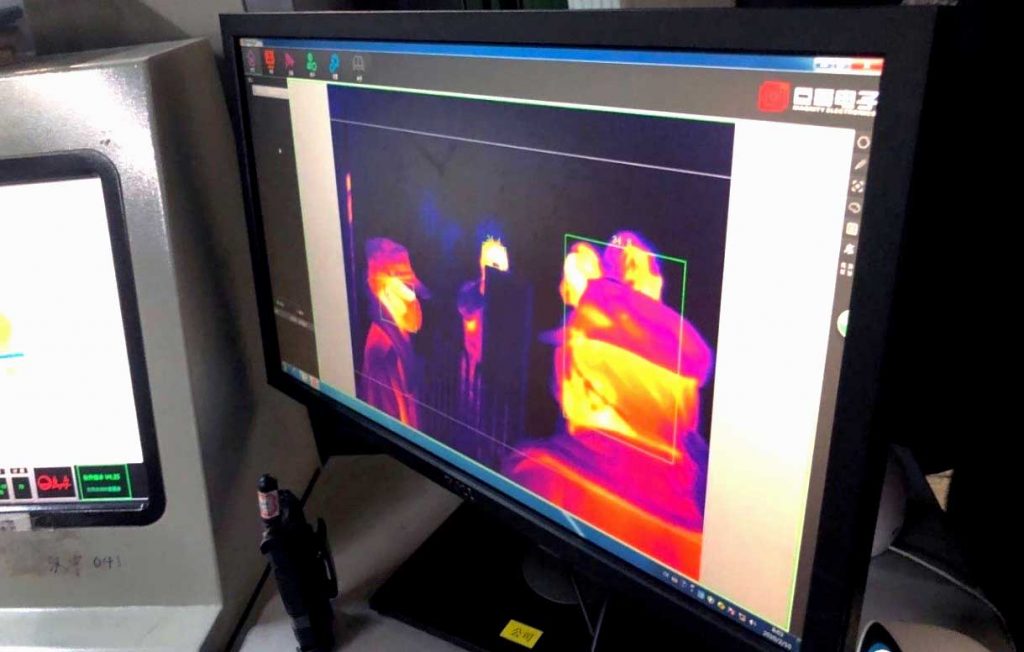
Masks are commonplace everywhere, and especially on the train. Some are taking extra precautions beyond what is recommended, including wearing gloves to prevent contact transmission. Rides can be quiet as people maintain social distancing.
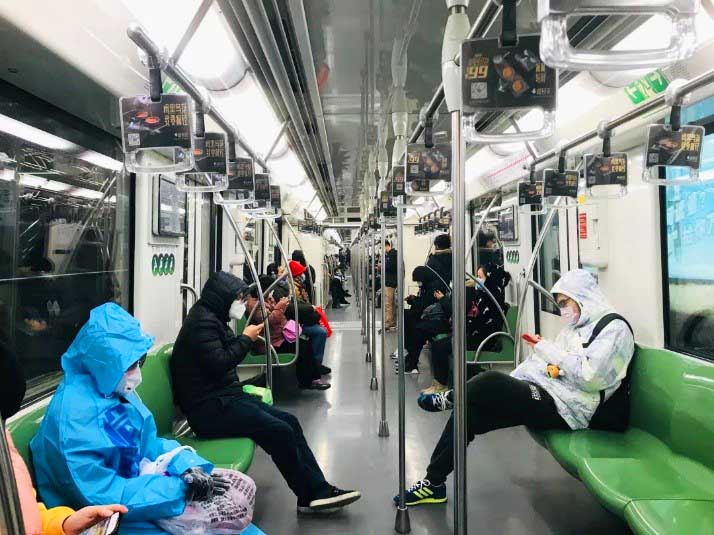
Ride-shares also remain a common way to commute. To provide protection for both driver and rider, most cars have been outfitted with plastic dividers that keep them separate. Masks and gloves are generally necessary here as well, despite having the space to yourself.
To receive Sustainability Matters and articles like this in your inbox, Sign Up Here
Upon reaching our office, security again scan your temperature as well as a QR code on your phone alerts you to whether you have recently been in contact with someone who has tested positive for COVID 19. While this “Safety Code” is an effective way to contact trace, it is an extreme measure that has brought up questions regarding individual privacy and civil liberties – with lingering concerns over what else can be done with the collected data. Green means you are healthy and cleared to enter the space. Yellow and red mean you must enter a 7-14 day quarantine. The massive adoption of this method allows authorities to trace movements of individuals and gain a quicker understanding of where that patient as been and identify others with whom they have been in contact with.
Entering the Office
Under our own office policy, whoever travels back from other Provinces throughout the country must self-quarantine for 14 days before they can return to our space. Our remote work capabilities have been well established at this point, allowing this extra protective safety measure for our staff.
We’ve instituted a Shoe Shelf. Our staff keep a pair of shoes in the office and change when they arrive. This is a common practice in a lot of cities where walking, biking, and public transit are primary methods of commuting. What was once done out of comfort is now an added health and wellness measure. Staff also change from street to work clothes once they enter the office.
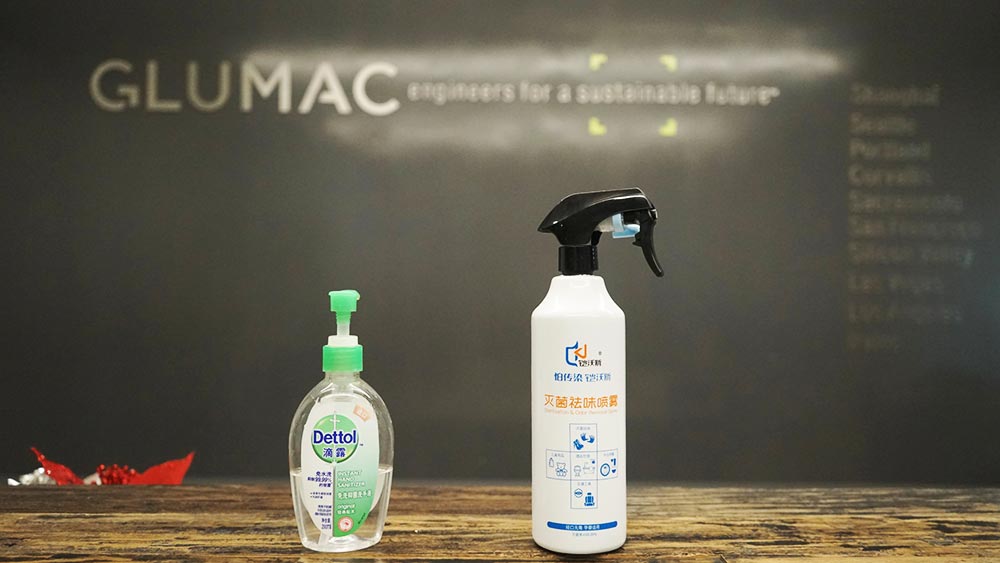
Hand Sanitizer and disinfectant are kept at the reception desk for employees to wash hands and disinfect before they come into the workplace. Advanced cleaning measures are necessary for surfaces as well. A balance between the types of cleaners we use and their true effectiveness needs to be struck – we don’t want to have to lean on toxic cleaners with terrible environmental outcomes like bleach to maintain baseline health in our work spaces.
The office manager measures the temperature for our employees every day before they come into the workplace using a handheld FLIR tempscreen digital thermometer. This allows the office manager to maintain a safe distance, and while they have already been scanned prior to arriving into the building, this is an added health protocol that deepens a sense of safety and wellness for staff. New masks are distributed to our staff each day as well.
Contact-free delivery is the new normal. We have placed a shelf at front of door for the courier to place packages on, with instructions to call the recipient to pick up the delivery once they’ve left. Lunch is delivered this way as well, though, most staff are now bringing food from home – and seeing dramatic improvements in their personal cooking skills!
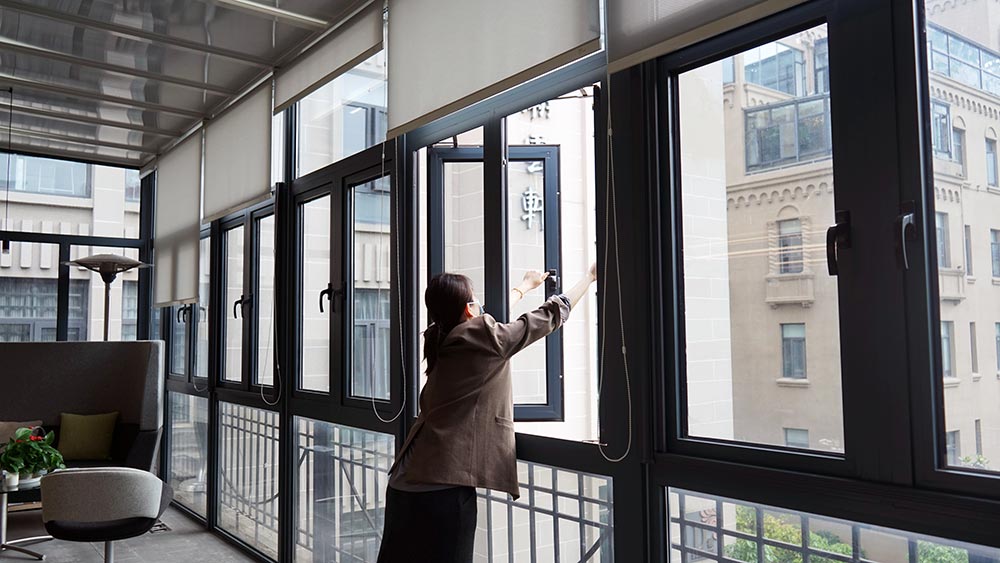
We have also installed three air purifiers throughout the space, they are kept on all day to reduce particles which may carry viruses. However, one of the best ways to maintain clean air levels in a space is to keep it well ventilated. This is something we’ve been working with clients on for years, but perhaps now more than ever the benefits are proving crucial. We turn off the return air and operate the AHU on Dedicated Outside Air mode to avoid cross contamination. This is one of our primary pieces of advice to clients. Completely shutting off return air is key, especially in high rise office towers where one AHU is serving for multiple tenant spaces.
The new normal looks a lot different. But these changes underscore the importance of sustainable and wellness-focused building design. As one of the most high-performance spaces in China, our space has already been equipped with or is easily retrofitted to integrate some of these helpful operational and behavioral changes to keep our staff safe.
If you’d like to learn more about these strategies, reach out to us at [email protected] to schedule remote presentation on strategies to mitigate the spread of COVID-19 in your workspace.
The recent pandemic caused many businesses to scramble to find ways to maintain productivity as stay-at-home orders from local and state governments all but cut-off traditional office spaces. At Glumac, we were fortunate to have practices and tools already in place that have enabled us to work without the need for developing new or untested procedures. This allowed our teams could continue to do what they do best without having to wait for new systems or tools to be available.
Whether its an abrupt change due to a public health crisis, or in an eased transition to remote work, there are a few things you’ll need to have in place to be successful: Existing expertise in cloud-based software; secure access; and an abundance of training. Here’s a look at what Glumac’s remote work transition looked like, and why it worked.
Leaning on Existing Expertise
This new working condition has given us the opportunity to hone our skills in various design collaboration tools. Many of our projects were already hosted on Autodesk BIM360. All of our users have access to this platform and other Autodesk tools, allowing us to scale usage with little downtime. The BIM360 platform allows us seamlessly connect teams in different locations – be it working across the city or the country – while working on the same models and files. Our work using this platform on the design of the State of California’s new Department of General Service’s building in Sacramento was recently profiled by Autodesk and demonstrates what can be achieved through this enhanced level of collaboration. Time spent in design becomes more efficient, with all parties collaborating in real time, mitigating rework and allowing for more focused time on innovation.
You can learn more about our net zero energy design work for the State of California by reading about our recent work on Sacramento’s Clifford L. Allenby Building
Because much of our staff were already well versed in the improved workflows allowed by BIM360, several potential initial pitfalls were avoided. Instead of having to spend time transitioning a large number of projects to the cloud, we were able to simply continue working, while increasing the number of projects in which we utilize BIM360. In fact, before our remote-work transition, Glumac was conducting 70% of our projects in BIM360. In the two months since, that number has increased to 90%.
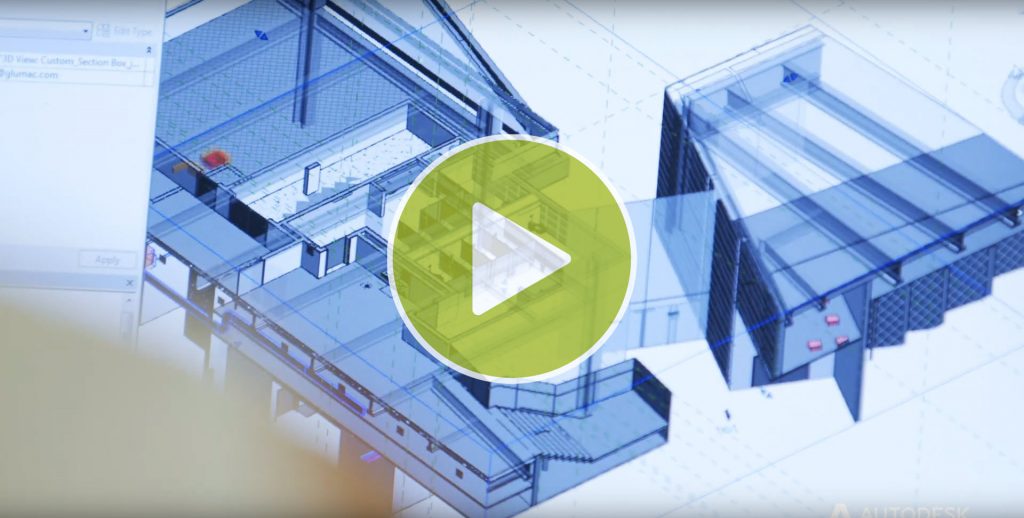
Secure Access
Our teams across the West Coast and Texas transitioned to a work-from-home operation essentially overnight. This abrupt but crucial transition was only possible because of the secure access measures already in place for staff. Secure VPN access to company servers and VDI virtual workstations on our network utilized by many groups and projects. Our IT infrastructure had already been set up with the ability for 100% of our US staff to have secure remote access to company servers to continue working on projects.
These systems survived the pandemic’s initial stress test, allowing us to protect the health of our workers and our company. Although, while we had collaboration practices and tools already in place, that doesn’t mean that evolving situations didn’t bring some changes. We have had to do things like monitor network bandwidth usage. Our IT department has been working to ensure users are continuously able to access services and that our infrastructure can adapt to the increased remote demand.
Training
Regardless of the speed of the transition to remote work, and the adeptness of your staff, training is necessary. Multiple training webinars have been held on tips and tricks for successful remote working and for taking advantage of collaboration features of Microsoft Teams and other applications. Our already extensive library of in-house training videos has now been made available to our staff via YouTube with some direct links inside of our Revit Tools, so users have even more ways to learn and increase their productivity while outside of the office. Glumac remains committed to innovate and looks for ways to improve our processes so that our engineering solutions and our ability to meet client needs continues to be as dynamic as the challenges that confront us.
“Real change occurs from the bottom up; it occurs person to person, and almost always occurs in small groups and locales, then bubbles up to larger vectors of change.” – Paul Hawken
The dynamics of the built environment are in constant flux – subject to the whims of emerging technologies, public health crises, and climate change. In 2020, more people than ever have begun to feel the squeeze of these issues, and the discussion around resiliency has become reactive to events directly affecting our daily lives. COVID-19 has grounded businesses, schools, and strained our healthcare system beyond the brink. Wildfires ravaged entire continents for months at a time. In response, we’re seeing a concerted effort at the design table that addresses these issues in the built environment. The tools are now coming online to demonstrate and integrate the resiliency measures necessary to create a sustainable future, but the onus remains on the A/E/C community to advance these measures as a matter of standard practice.
It’s a well-known fact – buildings generate 40% of the planet’s annual greenhouse gas emissions. What’s more, according to Architecture 2030, the global rate of new buildings constructed is expected to grow the size of an entire New York City, every month, for the next 40 years. In order to even come within hailing distance of our decarbonization goals, these buildings must operate at net-zero carbon. And to do that, we need to think about carbon more holistically. This means minimizing energy use, utilizing all-electric systems to avoid combustion emissions that come with burning gas on-site, and tying in renewable generated power. But beyond emissions, we need to consider the carbon impact of materials that go into these buildings and select lower carbon alternatives where possible.
What follows are trends we see across the design industry that have real potential to deliver a low carbon future.
The Convergence of Advocacy and Sustainable Design
It’s been two years since the landmark Intergovernmental Panel on Climate Change (IPCC) dropped like a ton of bricks its Special Report on the impact of Climate Change. And while the A/E/C industry isn’t the only one racing to meet the incredibly ambitious benchmarks necessary to avoid the worst consequences of climate change, 2020 is the year we need to move past merely recognizing the incredible impact our work has on global emissions and take more direct action in our communities.
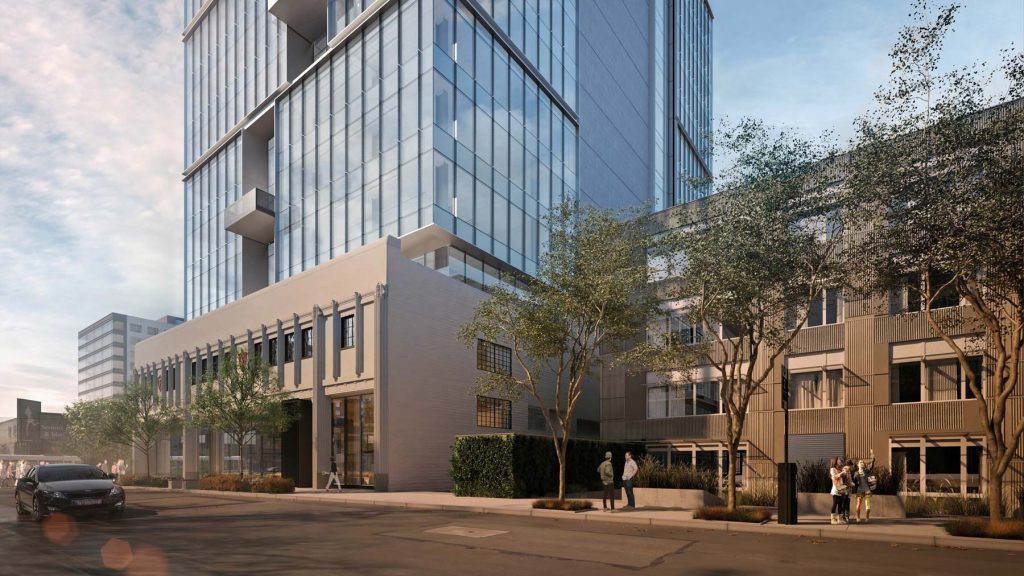
Global climate marches and youth activism have amplified the urgent need for governments and businesses to address climate change strategically and meaningfully. The resulting momentum is changing public opinion and has given the sustainable design industry a leadership position in this effort, as building developers and owners are increasingly requesting expertise for how their projects can satisfy climate goals. At the same time, building design experts can capitalize on this momentum now by increasing our involvement in policymaking locally and regionally to accelerate sustainable development in our communities – while creating an exciting pipeline of work!
At Glumac, our Sustainability and Energy teams are working actively with policymakers and advocacy groups to create the conditions that will facilitate major change. We’re working to develop a Living Building Pilot program in Portland, similar to the program already launched in Seattle, which incentivizes renewable and regenerative building design. And In Seattle, our energy group is working with the State of Washington to push what’s already considered one of the strictest building design codes in the country to enforce greater levels of sustainability and resiliency in new and existing buildings. And our Los Angeles energy group is working with the California Energy Commission and its state-approved software development team on revisions and enforcement measures to California’s energy code. They are also working with the state’s investor-owned utilities (PG&E, SoCal Edison, etc.) and Department of Energy on creating test procedures and efficiency standards on various HVAC equipment.
In the materials world, our architectural partners are leading the charge. One area of designer-led advocacy is manufacturers producing more Environmental Product Declarations (EPDs), largely in response to requests for greater transparency into the carbon impact of building products. EPDs – a statement that provides a clear snapshot of the environmental cost associated with a given product – are a crucial tool that help us make more informed design decisions and lead to more low-carbon outcomes. But they’re still not universally available, so it falls on designers to continue demanding them, not just for transparency’s sake, but also to create a market of better products. BuildingGreen’s letter calling for more sustainable products from mechanical, electrical, and plumbing manufacturers is a good example of how to do this. It’s providing firms a method of engaging directly with manufacturers and providing them with the specific product gaps designers need filled before they can take the necessary leaps forward in low carbon design.
The incredible minds within our industry hold the answers to these major problems we face. But it’s only through this kind of direct advocacy that any kind of meaningful change will happen. The path to mitigating climate change is a hard one to cut, but the meaningful steps are beginning now.
Embodied Carbon is the New High-Performance Frontier
Performance has always been associated with a building’s energy usage and water usage – and is generally measured by how well it conserves resources. But climate change has altered that definition. A building’s carbon emissions are now the most vital metric in determining its performance. And the most effective path to achieving this new level of high-performance building design is through lowering its embodied carbon.
The tools to accomplish this are now coming online, thanks in large part to building industry experts forging ahead on their own and creating ways to identify building materials that offer significant embodied carbon reductions.
“The level of product evaluation now available to designers, manufacturers, and policymakers is making a low carbon future possible.”
One such example is the Embodied Carbon in Construction Calculator (EC3). The tool, developed by Skanska and several partners, calculates carbon emissions embodied within a range of building materials, and provides clarity in the connection between design and procurement’s role in lowering embodied carbon. Another example is BuildingGreen’s recently released top 10 innovative green building products for 2020. It’s an invaluable list: from products to replace blown insulation, to medium-density fiberboard panels made out of rice straw, to gypsum that uses 25 percent less water, its most touted materials present not just energy and carbon savings while in use, but also carbon savings from lighter production and manufacturing processes.
The level of product evaluation now available to designers, manufacturers, and policymakers is making a low carbon future possible. The next step is incorporating these tools into how we talk about building performance with real estate developers and owners, so they can better understand how their decisions impact the project’s triple bottom line.
The Urban Land Institute has created a document for real estate professionals to better understand and advocate for low-carbon materials, and the American Institute of Architects (AIA) – through its commitment to the 2030 Challenge – has begun promoting the Design Data Exchange (DDx), for designer-based metrics on carbon. Finally, the Sweden Green Building Council has launched NollCO2, which sets clear goals for reducing embodied carbon and construction carbon.
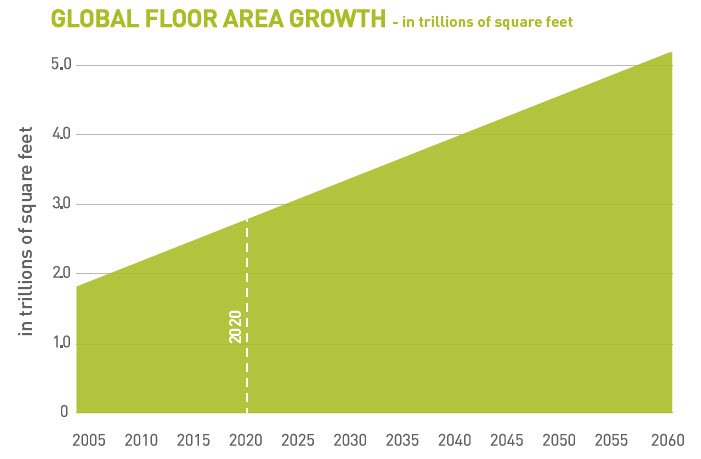
In our LEED material consulting work at Glumac, we have seen that it takes a pro-active approach to get lower carbon materials incorporated into a design, and then purchased and actually installed. But when we get it right, the project has an easier LEED documentation experience and can point to real improvements in the carbon performance and environmental impact of a new building. There’s even a new LEED v4.1 pilot credit for projects that demonstrate an embodied carbon reduction, without requiring a full Life Cycle Assessment. We are advocating for increased adoption of these tools (and others, such as the Arc platform that USGBC is using to track a project’s process to LEED Zero throughout this year and beyond. And judging from our conversations at February’s USGBC Town Halls on LEED Zero, we anticipate the industry at large will do the same.
Quantifying “Healthy Building Design”
Glumac has long-standing partnerships with the International Living Future Institute and biophilic design advocates like Terrapin Bright Green. Our sustainability team has worked with these organizations to educate the industry on approaches to creating spaces that perform efficiently and promote wellness for occupants. We’ve illuminated the human case: humans now spend more than 90% of our lives indoors, and it stands to reason those spaces should be promoting healthy outcomes. But there are still a lot of questions over how to quantify the business case, but to also quantify what a healthy building truly is.
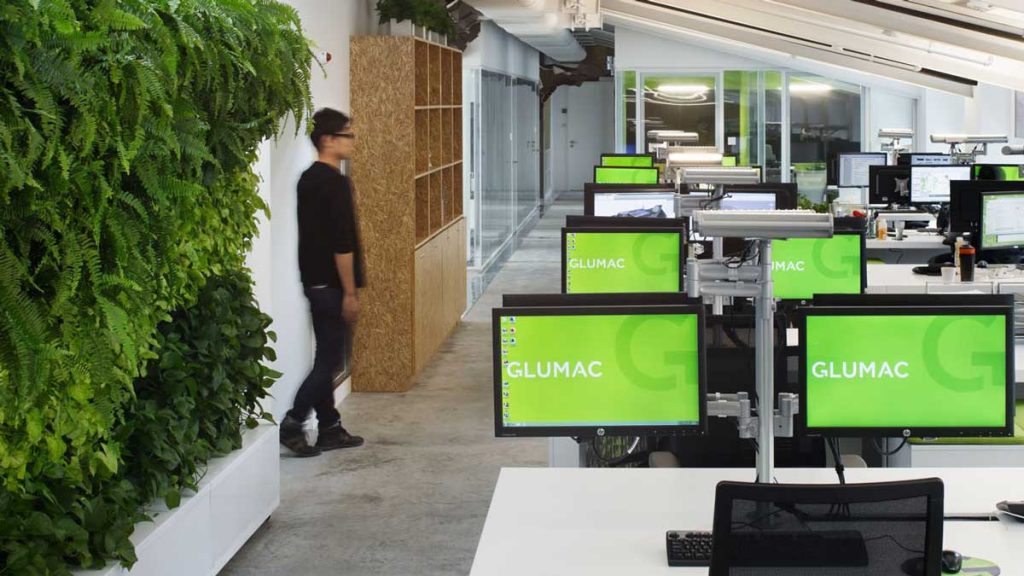
The technology to monitor and generate data is well beyond where it was 10 years ago, but we’re still trying to nail what the most effective actions are. The Arc platform is free and empowers us to gather uniform data on more projects than ever. And we can meter everything from CO2 to VOCs to levels of particulates in the air. But we’ve yet to develop a baseline of effective action to take with that data to improve the health of our buildings.
For more details on our scope of healthy building design experience, head over to our project portfolio at glumac.com
We are becoming increasingly aware of how the materials we choose impact the health of occupants. Flame retardants in fabrics is one area. San Francisco has already restricted the use of flame retardant chemicals in furniture construction in response to the negative health impacts such chemicals have on the people who manufacture and use them. But while we’re getting better at identifying what’s bad, the design industry’s ability to act on it remains in many ways a Catch-22. Improved products come on line, and Red Listed materials are becoming much more understood, but owners often feel they remain too expensive. But, the growing industry for these products is proving otherwise. The options are numerous, as seen on various materials databases such as UL Spot, Mindful Materials, and Transparency Catalog, and price is on par with conventional comparables.
Bringing Equity to the Forefront of Conversations
Social equity is a very local conversation that requires local knowledge and local partnerships, often with housing authorities, community groups, business associations, governments, and nonprofits. And there remains so much work to be done on all levels. We need better representation on design teams (e.g. in the a/e industry, the population of full-time workers who identify as women still remains under 20 percent), so our project decisions reflect the needs of diverse peoples. And as standard practice for any project, we need to reach out to these local partners to understand the diversity of communities in a place, issues of accessibility, and needs for services and amenities for public facing portions of the design. Often a more creative and dynamic design solution emerges from a challenge when it reflects the needs of people inside the building and the broader community who will interact on a variety of levels with the building on a daily basis.
“Equity is more than a topic of who benefits from design. It’s equally about how building emissions and storm and sewer conveyance will impact human health and quality of life in surrounding areas. “
But equity is more than a topic of who benefits from design. It’s equally about how building emissions and storm and sewer conveyance will impact human health and quality of life in surrounding areas. The environmental community is often faulted for neglecting the intersectionality between environmental issues and communities of social and economic diversity. Last year at Greenbuild, Enterprise Green Communities led a session alongside the International WELL Building Institute, titled “Using Health Data to Inform Design Strategies.” The organization spoke of the its efforts to integrate health into affordable housing projects, in part through its Health Action Plan with the USGBC. This is a positive step, with access to healthy and affordable housing still being a crucial need in essentially every city around the globe. But the understanding of how design choices impact our communities at large needs to remain at the forefront of the equity conversation.
This intersectionality is improving. “Environmental justice” is an increasingly useful way to tie together our environmental and equity goals. In Portland, a community-led ballot initiative led to the creation of the Portland Clean Energy Fund – a pool of funding that supports a lower-carbon future for citizens who are not usually part of development discussions. We’re also working with the Energy Trust of Oregon to study the cost-drivers associated with net-zero multifamily construction, and to develop resources design teams and energy modelers can use to estimate costs early in design, making it easier to optimize energy savings. Nationally, the NAACP’s initiative on Centering Equity in the Sustainable Building Sector will be a leader in making our equity goals a reality.
This type of hand-in-glove engagement with governmental and advocacy groups must continue to grow throughout the coming year.
Mass Timber is on the Rise – But Conservation Remains a Concern
Mass timber is gaining more acceptance as a more a renewable material and biophilic design choice. But it’s perhaps most notable as a building material with a very low level of embodied carbon. We’ve been working on a number of mass timber projects here at Glumac, most notably the First Tech Credit Union Headquarters in Hillsboro, Oregon. It presents a unique design challenge from an MEP perspective, but the results are as beautiful as they are sustainable.
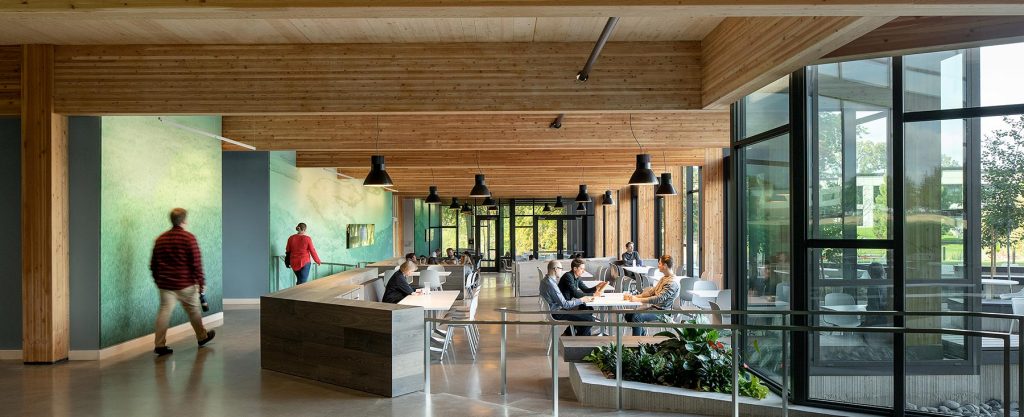
Whether for established products like Glulam beams or newer products like Cross Laminated Timber or Nail Laminated Timber, we are seeing more interest in wood construction for larger projects – this net-zero carbon neighborhood is a particularly exciting example. At the same time, communities have come to expect forests to provide a broad spectrum of benefits—economic returns, carbon storage, protecting water, a habitat for animals, etc. As more projects demand mass timber solutions in 2020 and beyond, we need to keep sourcing in mind to avoid unintended consequences of a new mass timber boom. One of the biggest challenges is finding ways to reward forest owners for their better management practices. Premiums for Forest Stewardship Council (FSC)-certified forest products often go to distributors or mills – while FSC landowners sell logs at market rate to end up in unlabeled products, and there are still very few options for certified mass timber.
This rise in demand means we all need to learn more about the “woodsheds” that feed into our building projects. Understanding where and how our products are sourced, whether certified or not, will help us deliver on the promise of this low-carbon building material, and while supporting ecosystems and rural communities.
Expanding Opportunities in Resilient Design
In recent years, the building design community has seen the conversation around “green building design” shift from “sustainable” to “resilient.” Sustainability was about finding balance between use and conservation. More and more, resiliency has become a response to climate-related scarcity and crisis (e.g., drying watersheds, wildfires, public health crisis, and flooding). It should still be seen as an opportunity to design built environments that operate more sustainably during normal conditions. Age-old passive design strategies like daylight harvesting and natural ventilation reduce energy demand, but can also keep buildings habitable during a prolonged outage. Or, divorcing buildings from local infrastructure by, for example, designing a building to store and treat solid waste onsite can allow it to operate if municipal systems no longer function. Adding these features to existing buildings can have a long payback period, but bundling these strategies as resiliency upgrades can improve the financial case for deep retrofit, as they pale in comparison to the potential cost of a damaged building or closed business.
“Our ability to model and synthesize data that projects future impacts and to demonstrate system effectiveness is well beyond where it was a decade ago.”
There is no one baseline solution, however. So the key to resilient design is being hyper local. Building professionals must break out of their business-as-usual designs that merely meet state and local code and instead embrace ones that address the risk of their local conditions given climate change and risks to public health. These conversations need to intensify at the design table throughout 2020 to make sure projects begin meeting the audacious goals ahead of us to avoid climate catastrophe. However, our ability to model and synthesize data that projects future impacts and to demonstrate system effectiveness is well beyond where it was a decade ago. And we can expect that to continue improving at an exponential rate.
Glumac’s California energy modelers are using several software applications that take into account future climate projects. Cal-Adapt provides data and visualizations that create a variety of climate change-related scenarios and allows us to provide more insightful and climate-focused design recommendations to clients. We’ve also begun working with Ladybug, a suite of open source tools that use data from the IPCC, the National Oceanic and Atmospheric Administration, and others to provide projectable climate data to create models that demonstrate everything from the changing effectiveness of renewables to the down-line impact on occupant comfort.
These are some of the tools that have already come online and are presenting a much more clear-eyed view of how climate will impact building design in a variety of geographic areas. The next step is demonstrating to clients the long-term value of the up-front investment. But these advanced modeling tools are helping us paint that picture more clearly.
Looking Forward
Our industry is a major contributor to the carbon problem. But the good news is the opportunity lies within us to create the lasting change necessary to drawdown carbon and deliver a sustainable future. By pushing harder for low-carbon materials, by being active with local and regional governments to advocate for stronger regulation, and by doing the hard work of delivering these solutions in an equitable way, we can get there. And the time to act is now.
To learn more about Glumac’s ongoing commitment to sustainability, check out our latest Corporate Sustainability Report.
The site of Creekside Community High School is less than a half-mile away from the Tigard High School in Tigard, Oregon. But the two facilities couldn’t be further apart.
Creekside–the Tigard-Tualatin School District’s center for students requiring a non-traditional path toward completing their education–has long resided in an aging schoolhouse wedged just off the driveway leading into the district. From there, students can see up the road to Tigard High School, its football, soccer, and baseball fields; tennis courts; swim center; and student theater. On site, there was a palpable sense that the alternative education path meant starting life on the outside looking in.
But Creekside is changing that. With the completion of its newest addition, the school will achieve net-zero energy-generating enough power on-site to offset, or even exceed, the needs of the facility. In an environment where students are in real need of inspiration, Creekside is now offering them not just a point of pride, but a facility that works to improve their education experience as a whole.
The Pitch: Inspire Through Design
The project’s primary ambition is to inspire. The students who attend Creekside are on the alternative education track. That is, students who require an approach to their education that is augmented from what is offered in a traditional public school.
To learn more about our education design experience, visit our project portfolio at glumac.com
The trouble is these schools tend to be afterthoughts – existing in repurposed storefronts; modular trailers; or, in Creekside’s case, an old schoolhouse. With a population of students in need of more attention and support to complete their education, the design of their surroundings can play a critical factor. By creating a space they can be proud to attend and that is outfitted with modern learning tools, their odds of success stand to improve.
How It’s Done: Simplify the Path to Net Zero
Designing for net-zero energy is often misunderstood as a lengthy and costly addition to the design process that complicates the delivery of otherwise high-performing buildings. However, complexity isn’t necessarily the key. While designing a facility to reach net-zero energy goes beyond a basic energy-in/energy-out equation, it has much more to do with mitigating energy needs from across the building’s mechanical, electrical, and plumbing systems. This means finding small amounts to take from each of these buckets, a piece at a time until the need is manageable by an on-site supply.
Continuing reading at trimtab.living-future.org
In the young history of building commissioning, we’ve already witnessed a surge of under-qualified firms that suffer from what I like to call the “I can figure that out” fallacy.
Yet, building commissioning is more than filling out and submitting paperwork to meet code requirements. It is a detailed process of analyzing complex building systems, ensuring they were installed correctly and are operating at optimal efficiency, and evaluating how the systems are operating holistically.
When conducted properly, building commissioning — be it retro-commissioning, re-commissioning or ongoing commissioning — can benefit building owners for many years to come. Expect lower operating costs, extended system and equipment lifespans, fewer maintenance or failure issues, and substantially more money in your pocket. That sounds good, right?
Learn more about our Building Commissioning experience at cx.glumac.com
But how do you know that you’re hiring the commissioning agents who will help you save the most money over the long haul of your building?
The following are some clear indicators that your commissioning firm is looking out for your best interests.
1. The Firm Is a Dedicated Team of Building Commissioning Agents
Many firms have designers who “switch hats” to offer building commissioning services when the need arises. The firms that will provide you the most value, though, have a dedicated team. These commissioning agents are knowledgeable on the latest industry trends, Cx tools and training, and are focused on providing expert commissioning services, nothing else. This results in a streamlined commissioning process that yields a more value-added commissioning service.
2. The Building Commissioning Firm Has Extensive Experience
It’s one thing to understand and implement the Commissioning Process on a project — it’s another thing to understand how the many building systems operate. Rarely do you find there is one commissioning agent who knows everything from mechanical to electrical to plumbing. You don’t want a mechanical commissioning agent commissioning your UPS emergency power system on your data center.
Continue reading at Tetra Tech’s IDMS website.
You may have had your building and manufacturing process undergo a thorough commissioning process when you designed and constructed your building. Good job! You are certainly enjoying all the benefits of building commissioning year after year after year — or are you?
When you buy a new car and the service light comes on, you wouldn’t dare not bring the car in for service. Like an automobile, you need to perform periodic tune–ups in order to keep your building and manufacturing systems running smoothly and energy efficiently.
Because once a building is in operation, the total energy use will increase as the efficiency of the building systems gradually decrease.
Re-commissioning your building systems every three to five years results in the past has proven the best way to keep your building systems operating at peak efficiency.
But with new technology, there is another option to consider — and it potentially could save you even more and extend your building’s life even longer.
Learn more about our Building Commissioning experience at cx.glumac.com
Instead of waiting up to five years to re–commission your systems, what if you could identify operating inefficiencies that lead to increase energy use as they happen, in real time? The building industry has been moving toward exactly this type of program by the name of ongoing commissioning.
What is Ongoing Building Commissioning?
Ongoing commissioning is a continuous process that tracks, measures and analyzes a building’s operational data over time, in real-time. This allows for building owners to continually operate their systems at high efficiency, thereby lowering energy use and costs and increasing the company’s profits.
The ongoing building commissioning process is a natural complement to the commissioning (or retro-commissioning) process, and it starts in the design phase of the project.
Continue reading at Tetra Tech’s IDMS website.
Fire suppression system? Check
Lane control signage? Check.
Traffic detection? Check.
From a deluge system capable of pouring as much as 17 inches of water per square foot, to the 300 cameras monitoring traffic, Seattle’s SR 99 tunnel might be one of the safest, most well-monitored spaces in the city. Traffic now passing below downtown is doing so in a tunnel designed to withstand a 9.0 earthquake and within seconds can alert drivers to anything from a multi-car pile up to a bucket that’s flown off the back of a truck.
But you don’t design and construct a mega-project like this and simply open it to more than 100,000 vehicles overnight. While ushering drivers from Point A to Point B (in this case, CenturyLink Field to the Space Needle) is any roadway’s primary objective, making that trip simple and seamless requires hundreds of MEP, lighting, low voltage, security, and digital safety systems – both inside and outside the tunnel – that each need to be inspected and tested. And tested. And tested again.
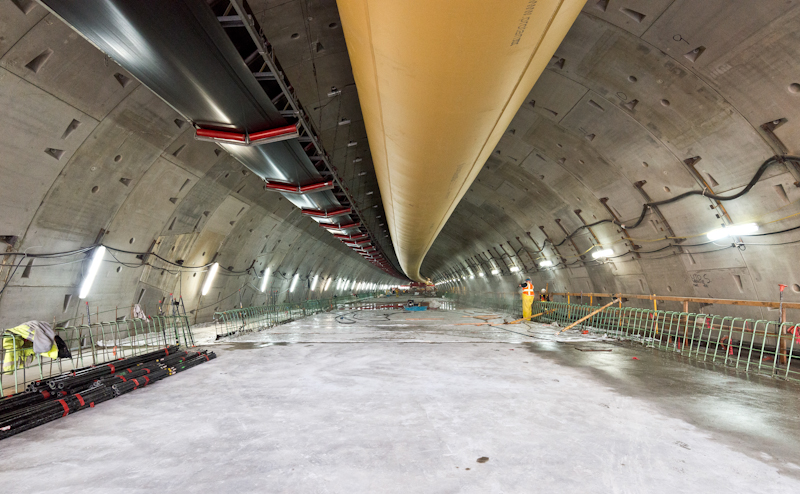
Glumac’s Seattle Commissioning Group, led by Angela Templin, performed functional testing for both the tunnel itself, and the operations buildings at each end. Beyond basic functionality, this type of project requires considering and testing multiple scenarios and iterations, to conclude as closely as possible the automated tunnel systems and the WSODT Operations staff are prepared to manage any problem that may arise when opened to the public.
“In sum, prior to the new tunnel receiving its first commuter earlier this year, commissioning agents need to test more than 100 individual systems and verify they are working both individually and together.”
Testing starts with every sub-system, then branches out from there to verify each system functions properly in conjunction with others. This means, for example, beginning by checking the drainage system to see if it’s pumping water where it needs to go. Then moving on to fire suppression – checking each of the 208 deluge values individually (both on the north and southbound side) to make sure they’re providing proper coverage throughout the tunnel. And because they had previously verified the pumps, the deluge water is now pumped out of the tunnel, preventing flooding. Then they verify lighting systems perform as intended for each emergency scenario (while also verifying lighting in the opposite direction of the tunnel maintains its normal, non-emergency operation). Then the PA systems are tested. Once all systems are tested individually, an emergency is simulated to see if each system functions seamlessly together to suppress a possible fire, alert drivers of the emergency, and give proper instructions and direction for escape if needed. In sum, prior to the new tunnel receiving its first commuter earlier this year, more than 100 individual systems needed to be tested and verified to be working both individually and together.
Commissioning in the cloud with CX Alloy
And all this is done with the daunting timeline of an incredibly visible project that causes intermittent freeway closures leading up to its completion and has a large, publicly-financed price tag attached.
The team needed a way to work fast, communicate easily, and to know the status of the ongoing testing. Historically, all the documentation on a commissioning project is managed and maintained within a project binder that contains all the documentation for every system. On a mega-project like this, the scale and number of systems make printing out and compiling massive binders of documentation nearly impossible – particularly when considering printing and materials cost as well as the physical space required. The time required for set up and ensuing back and forth communication between the Cx team, the contractor, and the owner risks setting the project back weeks, if not longer.
For more details on our scope of commissioning on the SR-99 Tunnel project, head over to our project portfolio at glumac.com
“On a massive scale you’re going from having a couple thousand pieces of equipment,” says Templin. “And a lot of systems in the tunnel come down to life safety. So, it’s critical to have that latest version of the documentation in one place accessible at any time by the entire team. The traditional paper binder would have significantly hampered the entire process.”
To accomplish that, the team worked together in cloud via Bluebeam and Cx Alloy. These tools allowed the entire team to work from the same information, mitigate rework, and built trust among the group, which is critical when so many systems boil down to life safety. What’s more, it provided greater efficiencies and ultimately sped up completion of the testing.
“Working in the cloud document repository allows the entire team to access that documentation in real time from anywhere,” Templin says. “Understanding the scale of this project, we pushed to collect a lot of documentation early on that we’d normally gather at the end of the project – testing documents, startup reports – all typically done as a close out. Because they were part of a pre-functional checklist, now that we’re near the end, 99-percent of those documents are where they need to be.”
Through this coordination-intensive project, the Glumac commissioning team has learned many lessons about the effective management of commissioning documentation, collaboration, and information sharing, and played a valuable role in verifying that one of the most high-tech tunnels in North America operates properly. Our team is always looking to leverage these lessons on our projects from a 16,000-square-foot community center up to the new North Satellite Terminal at Sea-Tac International Airport. Learn more about our building commissioning experience at cx.glumac.com.
Imagine a computer room or data center that was built before or shortly after the turn of the century, conceptualized without containment, and designed to a long-since abandoned practice of maintaining “meat-locker” conditions — a data center that never broke the barrier of 500 kW of IT load.
Most facility managers, if they’ve been involved with data centers at all, have seen such a place, and not at the turn of the century, but rather recently. Anecdotal evidence suggests that these “forgotten” computer rooms (or data centers) may have more installed IT capacity than all the hyperscale, super-efficient, highly publicized data centers combined. Whether this assumption is true or not, what we as an industry do know is that the opportunity to improve our nation’s data center efficiency remains a vast untouched resource.
For more details on our MEP engineering and data center design experience, head over to our project portfolio at glumac.com
Over the last 10 years, the data center industry has educated designers, operators, and managers on how to fix these inherent inefficiencies. As a result, facility managers now have many low- or no-cost ways to reduce energy use in the data center. (See below.) Most of these measures are well known. But facility managers may not be aware of one other very effective energy efficiency step that counts as low-hanging fruit and that is rarely addressed. That energy-conservation measure is an air-flow management strategy that can be summarized in two simple steps:
- Monitor IT loads in each cold aisle.
- Adjust the air flow delivered into the cold aisle to deliver an appropriate amount of cooling air for the load in that aisle.
There are many data centers that have been meticulously maintained over the years, yet they do not have an air flow management strategy to mitigate the results of re-circulation and bypass, which ultimately translate to hot spots, reduced system capacity, and wasted energy.
Read the full article at Facility Management Decisions Magazine
Glumac, partnering with Turner Construction, signed on to provide MEP engineering services on the fast-track, net-zero P Street design-build project in Sacramento, CA. Tapped to also bring its sustainability-focused know-how to the team, Glumac compressed the time-intensive workflows that are typically required to support projects with such audacious energy use goals. They did this by formulating a workflow that was equal to the ambition of the project, working with the project team from a shared Revit model using Autodesk BIM 360 Design software for design collaboration. This new level of teamwork helped boost productivity and condensed what are normally months-long project phases into mere days.
MEP Engineering for Green Buildings that Work
Glumac, which was recently acquired by Tetra Tech, has always had a commitment to designing building systems that efficiently support comfortable spaces. The firm has wholeheartedly embraced sustainable design, having designed more than 250 LEED-certified projects. Their eagerness to embrace innovation led Glumac to adopt BIM (Building Information Modeling) with Revit building design software before many other MEP engineering firms did, and the firm continues to forge ahead with its use of cloud-based design.
Ambitious sustainability goals come with an aggressive schedule
The Sacramento building will be the new home of the California Natural Resources Agency. Undertaken by California’s Department of General Services (DGS), to provide office space for more than 3,400 state employees, the P Street project is targeting a LEED Platinum certification and net-zero carbon use. The 22-story building will include 838,000 square feet of space. DGS decided to undertake the P Street building as a design-build project with an aggressive schedule for both design and construction.
The accelerated project schedule left little time between creating the high-level design concepts and developing the shop drawings the subcontractors would use for fabrication and installation of the building systems. That meant Glumac’s MEP engineers would have to juggle coordination with the architect and other engineers simultaneously as its team assisted the subcontractors—including Southland Industries, Redwood Electric, and Intech Mechanical—to develop shop drawings.
Continue reading on Autodesk’s Convergence blog.
Brian Stern is an energy analyst with Glumac, A Tetra Tech Company, who supports firm-wide sustainable and high-performance MEP Engineering and building design. His work improves building performance from initial concept all the way through operation. Using energy modeling software, he develops holistic building energy efficiency strategies appropriate to local environmental conditions. Brian’s focus spans all market sectors, having experience with the healthcare, commercial, higher education, institutional, residential, hospitality, and manufacturing industries. He is a graduate of the University of California, Los Angeles, with a Bachelors of Science in Mechanical Engineering.
###
What is the sustainable building design story of the Wilshire Grand?
The Wilshire Grand is really a holistically sustainable building. I think being such an impactful building on the Los Angeles skyline, the owners and AC Martin—the architect—wanted to make sure that the building had a strong, sustainability story and was really high performance.
We spent a lot of time during design making sure we could reduce the building’s impact on the environment, and the environment’s impact on the building. As Los Angeles’ climate is primarily cooling-driven—with a lot of bright, sunny days—reducing solar loads on the building as much as possible can make a major difference on energy efficiency. Our energy analysts and MEP engineers conducted building shading studies to determine solar loads for different sun positions on an average day and worked with AC Martin on optimizing the window-to-wall ratio, which is a reflection of what percentage of the building envelope—or facade—is made up of windows versus the amount made up of other constructed material. We also designed a really high-performance glazing for the curtain wall system.
For more details on our commercial high rise MEP Engineering experience, visit our project portfolio at glumac.com
The building also features a 550,000-gallon thermal energy storage tank that produces chilled water used in building conditioning. This system allows for the production of chilled water more efficiently at night and prepares the building to cool itself without drawing from city infrastructure during peak periods of the day, which helps to reduce the strain and impact on the grid. It also has a strong financial return for the owners, as the energy used to cool the building is greatly reduced.
The building also has a reclaimed water system that collects stormwater and condensate to replenish its cooling towers. Cooling towers use a ton of water—and being in Southern California and in a drought, this was extremely important to reduce the building’s impact.
Continue reading at Tetra Tech.com.
When the sustainable building design community discusses resilient buildings, the dialogue is often couched in phrases like “onsite energy independence,” “future-proofing,” and “community readiness.”
Yet, as we’ve learned from the increasing incidence of traumatic climate-related events, resiliency goes beyond keeping buildings upright and lights on. We have seen (and some of us may have unfortunately experienced) the consequences of severe wildfires, flooding, and storms that in 2017 alone cost the U.S. economy $312.7 billion dollars (NOAA 2017) in property and infrastructure damage, business disruption, and community displacement. More often the causes of these events arise from atypical conditions—draught and unusual weather—that collide with the seasons, sparking wildfires and unleashing tornadoes, hurricanes, dangerous winter weather and flooding. When the power goes out, the water isn’t safe to drink, and people can’t go anywhere, the risks and costs to businesses escalate minute by minute. Those repercussions aren’t limited to the communities affected. The media stories of the physical and mental stress the communities endure are felt globally, and the resulting emotions effect our daily lives, interfering with our relationships and hampering our productivity.
To learn more about our sustainable building design experience, visit our project portfolio at glumac.com
The frequency and intensity of weather-related events is growing. This is true, despite global and local efforts to reduce greenhouse gas emissions, which largely stem from fossil fuel use, as well as the more recent spotlight on meat consumption and food waste.
With all this happening and our knowledge of our impacts to the planet, it seems we need to re-evaluate our efforts in the building industry and rethink “high performance.”
Resiliency as an Indicator of High Performance Building
When we think of high performance buildings, the building industry has traditionally equated this term to something akin to “smart resource use,” and “energy efficiency” most often comes to mind because reducing fossil fuel use is the primary goal.
Continuing reading at trimtab.living-future.org
-Originally published in the 2018 Facade Tectonics 2018 Annual Conference & World Congress Conference proceedings, Vol I.
INTRODUCTION
Green building design is continuously evolving to require a more holistic approach, especially with ever escalating concerns about climate change, rising CO2 levels, and progressive energy code cycles. California’s Title 24 Energy Code is moving towards net-zero energy for all new construction commercial buildings by 2030. This push in sustainability and efficiency makes it increasingly important for architects and engineers to collaborate early in design to ensure that the architectural intent of a building can be maintained while effectively meeting energy code with the project’s sustainability goals.
BACKGROUND
The Hollywood & Gower high-rise residential project is in the entitlement phase of its project life. HKS started the design process with conceptual ideas that maximized views and efficiency while minimizing costs. The team needed to understand how the various shading designs would reduce solar gains in the units, ensure a comfortable environment with a high window-to-wall ratio, and identify if the design would pass energy code. The intent of this analysis was to understand the quantitative benefit to designing external shading for a building. We attempted to understand first how design decisions impact sustainability and compliance on the project. Then, through the lens of the energy model, we wanted to help inform future design decisions for the project.
METHOD
Using Hollywood & Gower, a high-rise residential project, as an example, this paper discusses how architects and engineers should work together in the conceptual and entitlement phases to optimize a building’s design and ensure the design intent can meet energy code. In a more traditional approach to analyzing the energy in building, the architect brings on the energy analyst in the beginning of the schematic design phase, or once the project design requires compliance documentation for city approval. The former option carries less risk than the latter as there is still an opportunity to influence the design to increase efficiency earlier in the process. In the Hollywood & Gower scenario, the architect and energy modeler worked hand in hand to analyze different envelope options. The following stages detail the design processes and analysis phases from the initial ideas to preliminary compliance modeling results.
STAGE 1 – DESIGN IDEAS
This proposed 22-story residential/mixed-use tower, located at the intersection of Hollywood and Gower, is generally regarded as being at the eastern gateway to the Hollywood Walk of Fame, where Lucille Ball’s star is at its front door. Its next-door neighbor, The Henry Fonda Theater, is a historically significant building and has hosted numerous high-profile live events. The tower, which contains 220 apartments, is designed for the young entrepreneur, or soon to be famous, stylish and hip-society type.
The tower is positioned on a north south axis to maximize views both to the north and the south for all units. To the north is a direct view to the Hollywood sign and to the south the Los Angeles city basin. On a clear day (yes we have them), the Pacific coastline and Catalina Island is visible. The orientation of the building also creates an opportunity for a sun-filled open space which acts as a “massing buffer” from the adjacent Fonda Theater and maximizes daylight entering the residents’ amenity space.
Several elevational/skin concepts were developed using Maya, a modeling, simulation and rendering software, for its ability to create rapid prototype concepts, each with an approach to creating a sustainable and performative skin that would preserve views and daylight but also protect against solar heat gain and glare.
Scheme 1 was developed with the notion of connecting the building to the larger street-grid, scaffold supports and intense shading blurring the boundaries of the building edges. Rendering courtesy of HKSScheme 2 took a far more measured approach and created a thin veil of metal tubes running horizontally, like venetian blinds only to be pierced by balcony openings. The second skin would extend beyond the footprint of the tower to emphasize the “superficial” nature and clearly identify the shading technique. In Scheme 1 and 2, the tower façade concept extended down to the podium levels tow rap and concealed parking and other uses.
Scheme 3 utilized a series of horizontal “trays.” Between each floor tray is a fluctuating ribbon of vision glass, patterned glass and metal panel, creating a digitized pattern. Vision glass is located to maximize exposure into living spaces, while bedrooms were primarily glazed with patterned or screened glass.
Scheme 4 established a series of shingled/angled glass panels in an attempt to create a skin resembling elements found in nature. Glass angles were set to maximize views and minimize solar heat gain. Panels on upper floors shaded the floor below and balconies could be added into each “cell” by removing glass selectively.
Probably the most striking feature of the tower will be its performative exo-skeletal bris-solei. Wrapped in a prefinished white aluminum panel, it was designed in hopes that it would:
- Maximize vision glazing around the building
- Create a foil which organizes the otherwise randomness of the “diving board” balconies. The skin is offset four feet, to create usable but structurally achievable platforms from the main structure in front of clear vision glass and dark metal mullions/panels. In a sense, the bris-solei becomes art and recalls the history of billboards, scaffolds and the structure of the iconic Hollywood Sign.
Full-length south-facing terrace balconies provide ample shading while not compromising views from the units. The rooftop features a full indoor prep kitchen, indoor activity room and landscaped terrace with outstanding views.
STAGE 2 – PRELIMINARY ENERGY MODELING – ARCHITECT
As described in the preceding section, the basic massing of the building and the options for shading structure – exo-skeletal bris-solei, were narrowed down based on various site parameters and restrictions, including view preservation strategies and aesthetics. Although it was obvious that any type of shading structure would have some positive impact on the energy consumption, it had not been quantified to help in the decision-making process. It was also important to determine how it would compare against a code-baseline building to make sure that it would meet code.
As a first step, the architects went through a rudimentary energy modeling exercise to quantify the comparative benefits of the selected shading strategies compared to a code-baseline building with no shading device. Additionally, this exercise informed the sensitivity of the window-to-wall ratio (WWR) to the performance of the building. The goal of the exercise was to identify a window to wall ratio for the design case combined with the shading structure that would meet the performance of a code-baseline building with no shading device and a 40% WWR.
The representative floor method was used to run energy simulations for the purpose of this exercise. A total of three floors were modeled. However only the results of the middle floor were used for calculations as the floors on the top and the bottom were only modeled to capture the boundary conditions, as would be the case in a typical floor in the residential tower. The model was created in Rhinoceros 3D and Honeybee for Grasshopper was used to run the energy simulations. Honeybee uses Energy Plus as its simulation engine. Although most of the geometry was created in Rhinoceros 3D, the windows were generated through Honeybee, which made it possible to control the WWR parametrically as a variable input.
The program of a mid-rise apartment was used to generate the default Honeybee model inputs for the simulations. The envelope parameters that were used are listed below:
- Roof insulation: R-27
- Wall insulation: R-8.7
- Window: U value 0.42; SHGC 0.28
Three sets of iterations were run, each with three variations. The first set was with no shading devices, with a window-to-wall ratio of 78%, 60%, and 40%. A 78% WWR was chosen as a maximum allowable. A 40% WWR is the ASHRAE 90.1 and Title-24 code baseline percentage that all energy models are compared against. The second set included horizontal shading devices with the same variations in WWR. The third set had the external frames as shading structure with the same variations in WWRs.
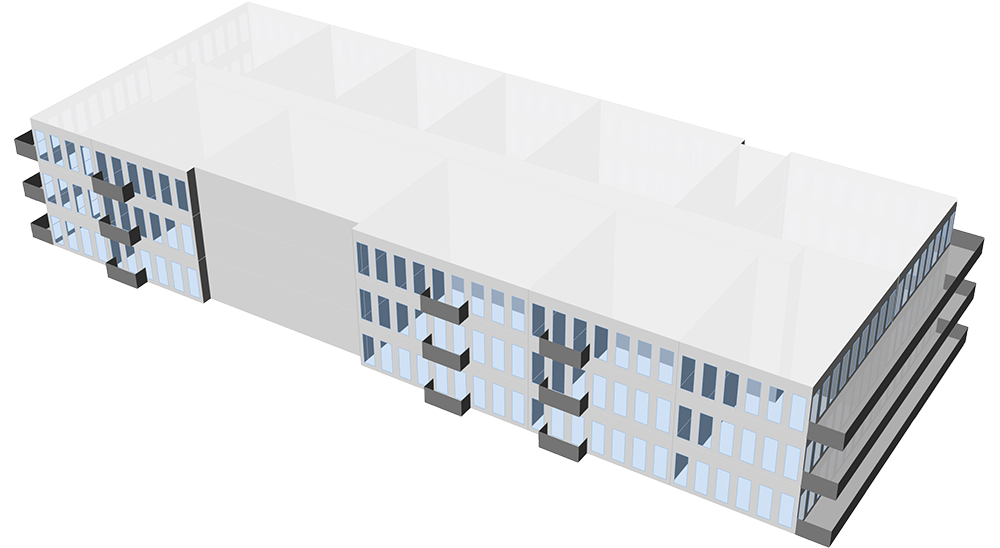
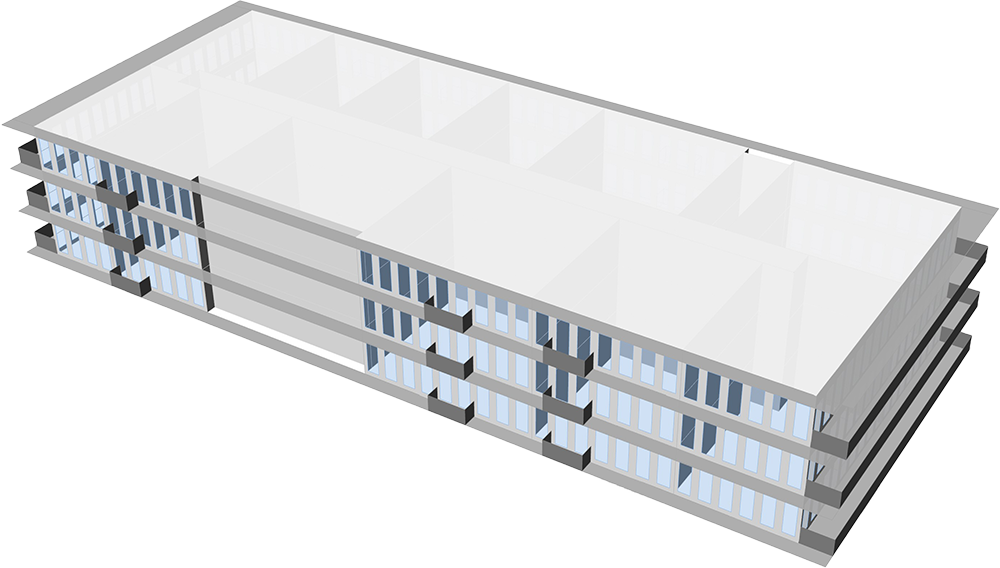
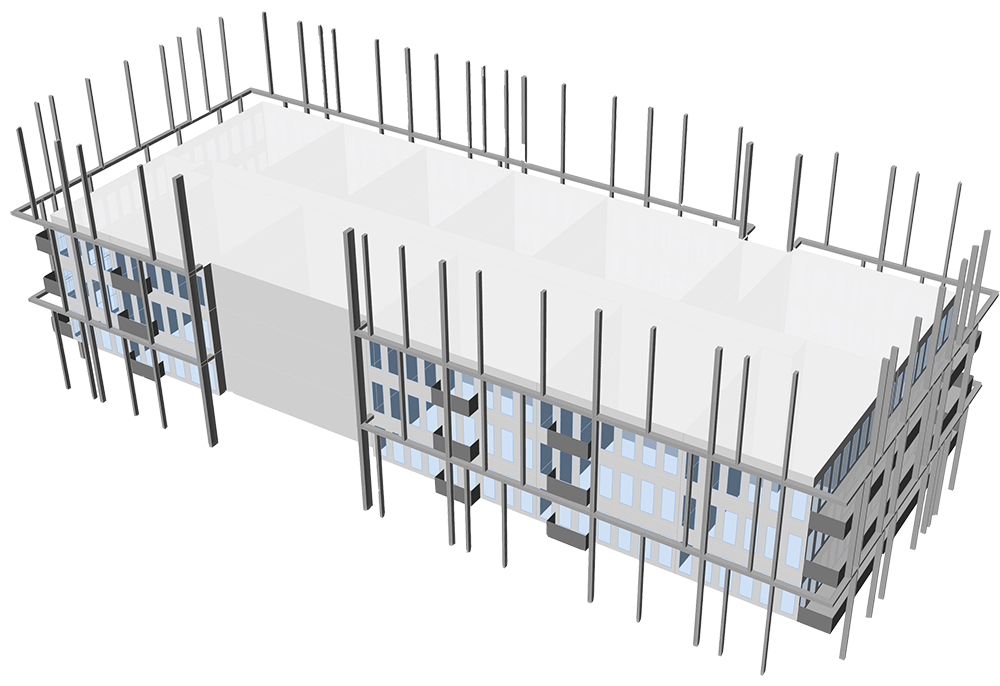
As the inputs for the electric light and equipment were kept constant, the results for those end uses show no change. The climate at the project site in Los Angeles (ASHRAE Climate Zone 3B, Title 24 Climate Zone 9) requires very little heating over the year, but has significant cooling needs. Thus, the load that the shading devices and the windows can impact the most is the cooling energy.
Isolating the cooling energy numbers, as shown in Figure 9, the option with shading devices at a WWR of 40% performs the best among the nine options, whereas the option with no shading at a WWR of 78% performs the worst.
The option with no shading device at a WWR of 40% was established as the baseline. With both horizontal shades and the frames as shading devices, the building could increase the WWR to about 60% and still perform better than the baseline (a percent savings of 1.7% and 3.5% in Table 1). However, the option of using frames as a shading device would perform better among the two. As the building has an elongated north-south orientation, and the east and west facades have maximum exposure, vertical shading elements of the frames at regular intervals proved beneficial to block solar radiation.
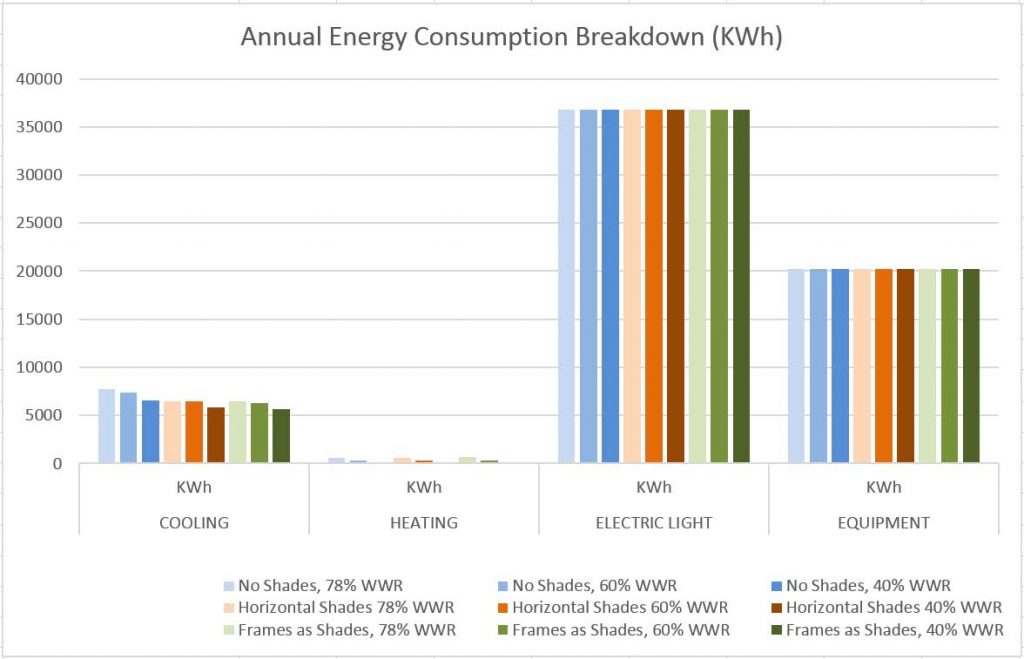

STAGE 3 – DETAILED ENERGY COMPLIANCE MODELING – ENGINEER
After the initial modeling effort, the team needed to see how the building was expected to comply with California Title-24 energy code. The team analyzed how the energy compliance margin was affected by different window to wall ratios and differing levels of exterior shading in the compliance model.
The project team modeled a three-floor representative section of the building. Using multipliers for a standard floor plate the energy performance of the entire residential portion of the building can be accurately extrapolated. The energy model was initially created in Revit to capture the full 3D geometry of the building. This was imported into CBECC-Com, the official state approved Title-24 compliance software. Based on previous experience with high-rise residential projects in Los Angeles, the team recommended that the representative residential portion of the building have a compliance margin of at least 3% better than code baseline.
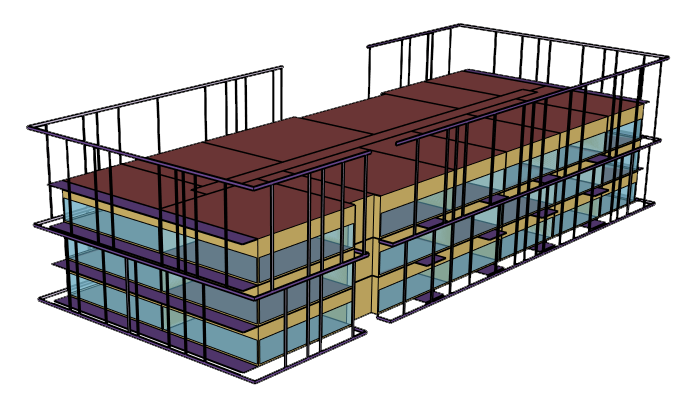
The project team assessed the shading structure with various window-to-wall ratios in order to determine its effect on the energy code compliance. Three different window-to-wall ratios were analyzed, 40%, 60%, and 78% to match the preliminary energy modeling analysis. Each WWR was analyzed both with and without the shading structure for comparison and to see its effect on compliance. This assessment aims to determine how impactful the structure and WWR are on energy code compliance.
The following outlines all assumptions that were assumed as part of the basis of design for the Hollywood & Gower. These parameters were based on past project experience with high-rise residential towers in Los Angeles.
Envelope
- Exterior Walls U-factor of 0.122
- Roof insulation of R-30 insulation with cool roof finish (SRI 0.9)
- Window SHGC of 0.25 and U-factor of 0.42 (thermally broken frames)
HVAC
- HVAC was composed of central condenser water loop with water source heat pumps (WSHPs)
- Condensing boilers: 93% efficiency
- Closed loop fluid cooler with a 10F degree approach
- Dedicated outside air corridor ventilation unit (DOAS)
- Condensing DHW water heaters with 96% efficiency
- The residential unit WSHPs (17 EER, 5 COP) and ECM fan motors
The building was also assessed for three shading options: no balconies or cage, balconies only, and with both the balconies and the cage. The Revit geometry models of each are shown in Figures 11-13, and the compliance results of these simulations are shown below in Table 2.
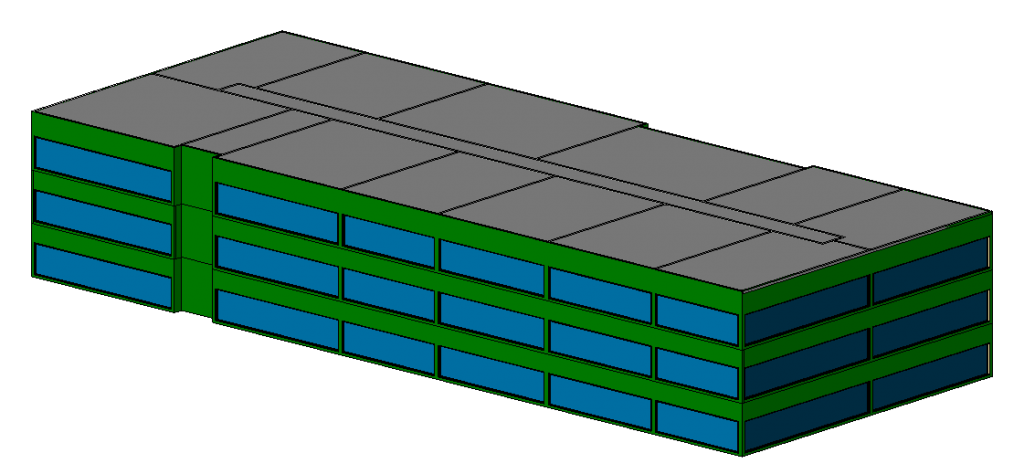
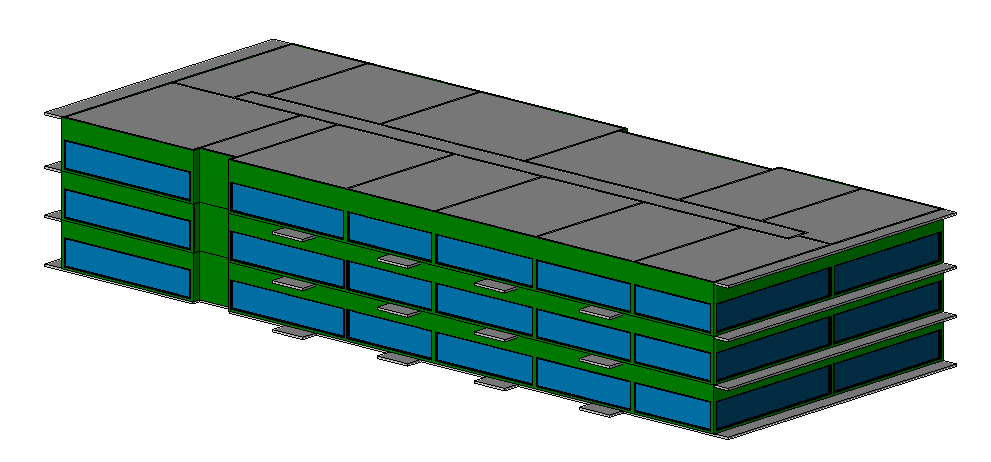
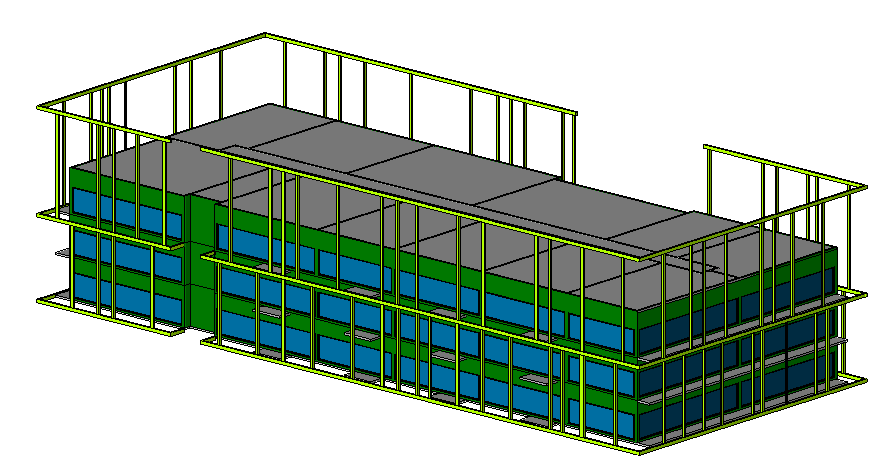
It was determined that that both the shading options and WWR had a significant effect on complying with Title-24. The 60% WWR with both balconies and the cage exceeded code by 1.2%, and would only require minor design changes to meet the recommended compliance margin.
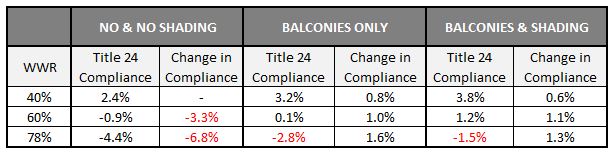
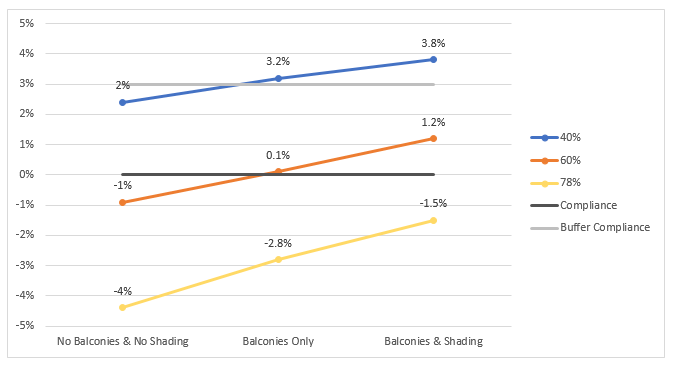
The 78% WWR design took a more significant compliance penalty of -6.8% relative to a baseline 40% WWR design. The balconies and cage shading structures were very helpful in reducing cooling-related loads and improved the compliance margin by 2.9%. This however did not make up for the overall penalty, and additional energy efficiency upgrades would be required to offset the increase in WWR, so additional energy efficiency measures are required in order to obtain the 3% target margin for a mixed use residential building. These energy efficiency measures could include high performance glazing, premium efficiency HVAC equipment, or a solar hot water system to offset the domestic hot water load.
EXPLANATION
The Honeybee and CBECC-Com models both show the same narrative, that an increase in window-to-wall ratio has a decrease in performance due to the increase in cooling loads. From this analysis, we can deduce that through the development of an energy model of the exterior shading of the building, the energy impact can be quantified and implications can be ascertained. By designing and modeling detailed exterior shading early in design, one is more flexible to create a more interesting building with a lower cooling load and larger windows. External shading design works best when it is validated both architecturally and by the design engineer in order to create an integrated design that adds to the building aesthetically as well as for energy performance. Without the integrated process, the systems will not be optimized for efficiency and could potentially add cost with minimal performance benefit. This type of early collaboration is critical to meeting sustainability goals such as Architecture 2030 or Title-24 Net Zero Energy.
CONCLUSION & FUTURE WORK
Because the architect worked hand-in-hand with both the initial energy modeler and the compliance energy modeler, every step of the external shading structure was analyzed. Performing these iterative energy analyses in the entitlement phase is critical. The entitlement phase sets a precedent for the project and creates some design constraints for the project moving forward. If the building design is floor-to-floor glazing in the entitlement phase, when the building goes through compliance the HVAC system will need to be able to mitigate the harsh solar loads and will end up increasing the cost of the building’s HVAC system.
The design of a 21st century building requires a holistic approach. Architectural and structural elements influence the mitigation loads required by HVAC (possibly saving on first cost) and can potentially lessen the energy requirements of the building. These up-front analyses help integrate different disciplines and assist in understanding the impact of design decisions early in the process. While this specific CBECC-Com analysis will resonate most specifically with people in California, the results and understanding span globally.
Moving forward, green building design will continuously evolve to include this holistic approach. With escalating concerns about climate change and CO2 levels in the environment, it is becoming evidently clear that even the highest standards of construction performance used today are not progressive enough. In order to hit Architecture 2030, it will not be enough to decrease the solar loads through the use of external shading. More passive strategies are needed, such as natural ventilation, night pre-cooling strategies, daylighting, solar tubes, designing with thermal mass, using perennial plants as shade walls, and optimizing solar penetration into the building for heating/cooling mitigation.
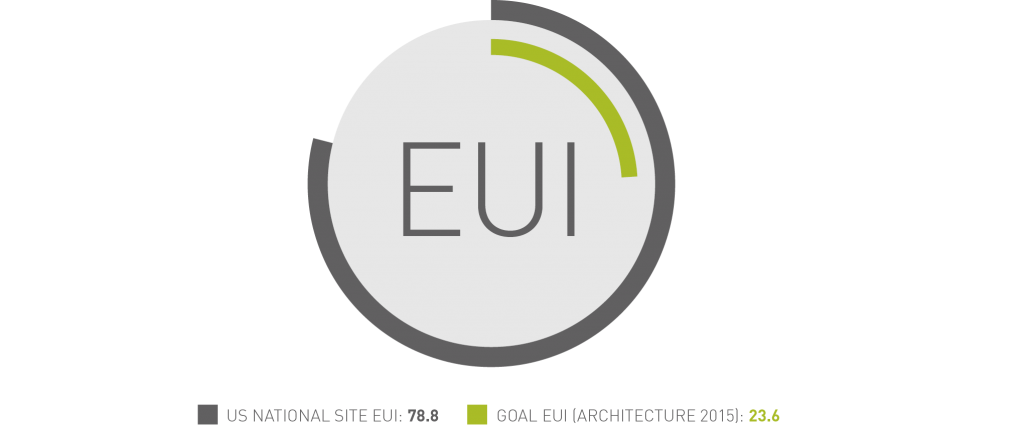
By optimizing the building for the climate in which it resides, the project can take advantage of “free” heating or cooling. These strategies can allow for reduced HVAC loads and can decrease first cost on HVAC systems and savings on utility costs throughout the project’s life. By tailoring the envelope and building design to incorporate natural lighting through daylighting or solar tubes, the project can decrease the amount of energy required to light the space while also providing a better environment for the tenant.
ACKNOWLEDGMENTS
Thank you to Brian Stern and Jacob Chan for their help with the analysis and report.
REFERENCES
Website: The American Institute of Architects. “The Carbon Neutral Design Project: What is Carbon Neutral Design?”
http://www.tboake.com/carbon-aia/carbon_definition.html (accessed August 25, 2017).
Website: Architecture 2030. “The 2030 Challenge”
http://architecture2030.org/2030_challenges/2030-challenge/ (accessed August 12, 2017).
Website: Ladybug Tools
www.ladybug.tools
The timber trend is beginning to gain traction as a potential change agent in sustainable building design – particularly in forest-heavy areas of the country like the Pacific Northwest. And it’s due in large part to the increasing availability of Cross Laminated Timber (CLT). CLT is a prefabricated material consisting of sustainably-sourced lumber layered in opposing directions and held together with low-VOC adhesives. The result is a powerful load-bearing material that is proving its mettle as a competitor to steel and concrete.
It’s causing many MEP engineers and sustainable building design professionals to come around to the idea that wood structures are a realistic sustainable approach. But what are the environmental benefits? According to a recent article in The Architect’s Newspaper, utilizing timber as a structural material reduces the associated carbon emissions of a building in three ways:
- Wood is a renewable resource with a lower extraction and processing impact than steel and concrete.
- Because it’s a profoundly available domestic resource, the embodied carbon in timber is significantly lower than that of steel or concrete, which more and more involve importing materials by ship from other parts of the world.
- The more wood we harvest, the more we can replant, increasing the land’s carbon sequestration capacity.
This final point may seem a little counter-intuitive, but substantial research is being done on the subject of a monitored increase in timber harvesting. It begins to make more sense when we learn that much of the wood harvested to make CLT is Mountain Pine Beetle kill trees – essentially trees that are dying due to a natural beetle infestation process and are beginning to decay and release more carbon into the air, rather than absorb it.
Coupled with emerging trend of mass timber structures is the interior design concept of exposing all building services (ductwork, chilled sails, fire protection piping, etc.). This idea has branched out of tech office space into a mainstream approach of designing a more industrial and functional indoor experience. By removing dropped ceilings, interiors feel more spacious. And exposing the wood structure creates an aesthetically pleasing and natural environment, as opposed to the more traditional concrete or steel.
Healthy Building Design is Sustainable Building Design
There are a number of ways these trends promote sustainability in modern work spaces. Our MEP engineering experts are continuing to investigate and learn the benefits of design approaches that value the health and well-being of the occupant. Pulling from the knowledge found in the WELL Building Standard, there are three relevant concepts that can contribute to the overall performance of a building:
- Beauty and Design
- Biophilia
- Material Transparency
According to the WELL standard, “integrating aesthetically pleasing elements into a space can help building occupants derive a measure of comfort or joy from their surroundings.” Presenteeism – the idea that while staff are present, they are often disengaged from work — is a major issue in office spaces. Thoughtful design and the use of natural materials can help combat this.
To learn how our MEP Engineering experts approach WELL Building certification, check out our
case study on Healthy Building Design
Biophilia addresses our affinity to the natural world, and through incorporating environmental elements and natural patterns, interior spaces can “help to speed up healing and recovery time, boost positive feelings and reduce negative ones.” This is of particular use in healthcare facilities, where biophilic design has demonstrated improved speed of patient recovery and leading to more availability for new patient intake, and office environments, where it has shown a reduction in lost time from staff fatigue.
Material transparency is the concept that we as building occupants should know what our buildings are made of, including the myriad of chemicals that are constituents of seemingly harmless components. With wood, the only component we need to be aware of is adhesives used to create cross laminated timber and glu-lam products. There are environmentally sound products for this application.
Sustainable sourcing combined with thoughtful interior design can lead to gains in productivity and energy efficiency. Our MEP engineering experts are continuing to investigate the sustainable building design benefits of timber and are already integrating it into commercial projects. As we move closer to a future of net-zero and net-positive design, the inclusion of natural material will prove critical.
Building commissioning has taken root. In the last decade, implementation of the building commissioning process has expanded greatly throughout the commercial market. This is due in no small part to the Lawrence Berkley National Laboratory’s landmark 2009 study “Building Commissioning: A Golden Opportunity for Reducing Energy Costs and Greenhouse-Gas Emissions.” However, the majority of building owners’ awareness still tends to come abruptly when a code official requests a commissioning report during inspections or prior to building turnover.
In this context, commissioning becomes another box to check, rather than a process from which owners can extract real value. Approaching commissioning in this way is a little like learning your car may have an issue with its brakes and just asking the mechanic to make sure your seatbelt is functional. Operating this way, we lose the value in letting an expert assess a system and recommend potential fixes so optimum performance can be achieved and prevent real issues from arising.
So instead of discussing how to simply check our boxes and move on, let’s talk about how we can change the perception of commissioning from a process that’s required to something that’s desired.
Value in Building Commissioning
We’ve discussed what building commissioning is – but what about the value for owners? As stated by the Lawrence Berkley study, benefits of commissioning include:
- Financial – The payback on initial commissioning is returned in as few as 1.5 years on new construction projects.
- Environmental – Building systems properly aligned and installed with the oversight of a commissioning team reduce CO2 emissions by about 340 megatons per year).
- Operational – Building commissioning agents provide operations and maintenance staff with detailed documentation that gives them the knowledge base to make sure the first two benefits are achieved.
As energy codes tighten, particularly in areas like Washington and California, these things are changing from fringe benefits to baseline requirements. To meet strict codes; to support the integrity of innovative engineering and architectural designs; and to ultimately net timely and realistic ROI, early and collaborative engagement with a building commissioning agent is crucial.
Learn more about our Building Commissioning experience at cx.glumac.com
Commissioning agents act as a bridge between the owner and project team. Ideally, they join the design team during conceptual design – helping provide checks to make sure owner’s goals are on track – and stay on through at least the first year of operation to provide operations and maintenance staff the tools they need to maintain continued high performance.
Issues Prevented by Building Commissioning
Glumac’s building commissioning agents regularly encounters issues that could go unnoticed if the project wasn’t going through the commissioning process. Examples include:
- A VRF economizer configured backwards in a mixed-use retail space, leading to drawing in too much cold air when the system is in heating. Glumac commissioning agents identified the issue during the functional testing process and helped the team troubleshoot the cause of the issue, reducing inefficiency, saving money, and improving general comfort, all before the building’s occupancy.
- Sump pumps with incomplete control panel connections in below-grade parking garages that could lead to a failure to notify the operations team a pump had failed or, worse yet, a flooded basement. Glumac has experienced this issue multiple times on a number of projects and the cause of the issue isn’t any one contractor’s fault. Instead, since the panels are typically ordered by the mechanical or plumbing contractor and installed with coordination between the mechanical, plumbing, electrical contractors and the pump and control panel vendors, it’s very easy to mistakenly miss one connection. The building commissioning process helps verify all parties have coordinated properly and the pumps run as required.
- On a museum office project, Glumac reviewed the design of the standby generator and automatic transfer switch. Our commissioning agents suggested performing testing with a total building blackout. The result was the discovery that the VRF system for the room and storage area was not functioning. The fan coil unit and outdoor condensing unit had power but were not running. Glumac assisted with a design change. Without total blackout testing, the project team would not have discovered this issue.
Achieving Desired Value Instead of Required Value
There are three takeaways here.
First, find out early if you’ll be required to have building commissioning on your project and understand what systems or equipment will need to be included. In the case of LEED projects, the LEED Reference Guide is very good at spelling out which systems are to be included. When it comes to energy codes, if the applicable section isn’t clear enough, there may be other organizations that can help you understand the required scope such as NEEC, or the AHJ may have helpful documents like Seattle’s CAM 419, which breaks down the Cx section of the code in easy-to-understand language.
Second, commissioning providers are your advocate. Their role is purely to make sure you’re getting what you need from a building, and they’re eager to help. Don’t be afraid to ask your commissioning providers what credentials or certifications they have and do some research to learn more about what each certification entails. This will help you feel confident that you’re getting the best advocate out there.
Finally and perhaps most importantly, don’t be leery of hiring a commissioning provider early, for fear of cost. Hiring building commissioning agents at the beginning of a project is negligibly more expensive than hiring at the last minute, as the deliverables and field work will be roughly the same. Plus, early involvement facilitates early identification of issues. Making a change in CAD or Revit is much easier than re-routing ductwork and conduit after it’s been installed. Additionally, through early involvement, the commissioning provider can help form a cohesive team, with a unified goal of delivering the best possible product from day 1 to year 100.
On a project, don’t buckle your seat belt and hope for the best. Embrace the building commissioning process and let it help achieve the best outcome possible.
In part one of this article, we discussed the financial benefits of using radiant systems, namely the cost reduction benefits while helping spaces achieve optimal occupant comfort levels. Now, we want to further examine the technology by examining its ability to maintain the integrity of architectural designs and improving occupant wellness and productivity.
Integrating Sustainable Building Design Elements with Architecture
Radiant systems offer a great deal of flexibility for both interior and exterior features. For example, while in cooling mode, the material properties of radiant slabs and panels allow them to absorb excess lingering heat, allowing for more effective conditioning. As the trend of glass-dominated building envelopes continues to surge, this proves particularly useful. In those environments various surface areas throughout the day will be affected directly by excessive sunlight. This mitigates the tug and pull of system needs and code challenges clashing with excessive glass.
Conversely, radiant heating operates in response to the gradual cool-down of a space. As a space grows colder, the radiant system can dissipate more heat accordingly. In this sense, radiant technologies maintain a building’s envelope materials at a temperature that optimizes comfort, rather than fighting against the effects of exterior weather conditions.
To learn more about our MEP engineering and sustainable building design experience, visit our project portfolio at glumac.com
On the interior, radiant can be integrated as an underfloor system, or via panels that can be designed to match patterns and textures of interior elements. A terrific example of this is our design of a two-story ballroom at the Kimpton Sawyer Hotel in Sacramento with wood-paneled ceilings and all glazing on the exterior wall. The design incorporates heating-only ceiling radiant panels that are designed to offset heat loss through the glazing of the windows. Because the ballroom has all glazing on the exterior wall, a primary concern during design was how to offset heat loss through the building envelope – a common issue in buildings that feature a glass façade. The radiant panels were placed to provide heat to the perimeters where heat is being lost through the glazing. The radiant manufacturers designed the ceiling panels to match the patterns and texture of the wood-paneled ceiling, making the radiant panels practically invisible to occupants. Hence the panels provide a comfortable environment while simultaneously maintaining integral architectural features.
Radiant systems are also easily integrated with other types of mechanical systems. Whether it’s designing a new central plant or trying to incorporate a radiant system into an existing system, there is flexibility when it comes to tenant options. For example, a central plant that serves a radiant system can also serve air handlers in other parts of the buildings or fan coil units.
Healthy Buildings Make Healthy Occupants
An emerging trend in sustainable building design communities is designing spaces that focus on occupant wellness. Be it a commercial office environment, classroom, or healthcare facility, MEP engineering experts are learning more and more about the positive effect bringing nature-inspired design into the built environment can have on an occupant. Glumac’s MEP engineering experts are increasing recommending radiant systems, which synergize with this approach very effectively, as they condition using a naturally occurring process. As the radiant effect accounts for a large part of our physical interaction with our surroundings, implementing radiant systems can dramatically increase occupant comfort in the built environment. For example, sunbathing in 60-degree ambient temperature can still be comfortable because of the radiative effects of the sun. Take away the radiative component, and you’re left with 60-degree ambient temperature and an uncomfortable environment. Radiant systems also help eliminate common comfort issues associated with traditional HVAC designs, such as draft and noise, due to the reduced size of the air-side ductwork and associated equipment.
Terrapin Bright Green’s study on biophilic design patterns suggests that implementing thermal and airflow variability positively impacts comfort levels, well-being, and productivity among occupants. This design pattern can be expressed by subtlety changing ambient air temperatures, relative humidity, and indoor airflow. Radiant surface materials can provide thermal variability in mimicking the effects of naturally occurring radiative elements, such as the heat felt from a stove or fireplace, or the cold felt from an ice rink. Terrapin’s study revealed that implementing these design patterns can positively impact occupants’ concentration and improved perception of temporal and spatial pleasure[1], which can lead to more motivated and goal-oriented behavior. Although occupant health and productivity is a concept not easily quantified, the benefits are evident. Increased occupant health can decrease health-related costs for employers, and increased productivity and concentration can result in higher-quality and more efficient work. Happier and healthier occupants make for a healthier and more cost-effective building.
In 2014, ASHRAE Journal published an article by Guruprakash Sastry and Peter Rumsey comparing VAV to radiant, based on the studies performed in the Infosys SDB-1 building in India. According to Sastry and Rumsey, surveys revealed an 18% increase in occupant comfort from VAV to radiant.[2] This case-study found that properly designed radiant systems are more efficient than VAV systems in terms of energy, occupant comfort, and cost.
Radiant technologies and their uses can be easily compared to earth’s natural systems. The sun is the Earth’s radiative heat source – a natural energy source whose effects we can observe and implement in the built environment through radiant systems. In our efforts to create a built environment that is healthy for humans, radiant is a practical and appealing solution that implements the important comfort aspect of a healthy building.
[1] Browning, William, Catherine Ryan, and Joseph Clancy. “14 Patterns of Biophilic Design.” Table 1: Biophilic Design Patterns & Biological Responses. Terrapin Home – Terrapin Bright Green. N.p., 12 Sept. 2014. Web. 07 Aug. 2017.
[2] Sastry, Guruprakash, and Peter Rumsey. “VAV vs. Radiant: Side-by-Side Comparison.” ASHRAE Journal 56.5 (2014): n. pag. Web. 7 Aug. 2017.
When Gensler began designing their new studio in downtown San Diego, they wanted a human-centric space. Taking over the former NBC Broadcasting studio on the first floor of downtown’s 225 Broadway Tower, Gensler wanted the space to be a showcase for innovative and sustainable building design. Designed to achieve both LEED Platinum and WELL Building Standard certifications, the new studio underscores the benefits and functionality of sustainable design strategies such as natural ventilation, radiant heating and cooling, daylighting, and circadian lighting design.
HVAC
Gensler’s vision for the studio included creating a pathway between the first floor retail-style space, with the second floor traditional office space. Because the prior tenant was the NBC broadcasting studio, code required that part of the first floor have ballistic glass. As a part of the renovation, the ballistic glass was removed and new, more energy efficient glazing installed.
One of the first concepts to emerge between Glumac and Gensler revolved around natural ventilation. Traditional design for heating and cooling systems recommends a very strict range of temperatures and humidity, set point temperatures between 70F and 75F, and relative humidity between 30% and 50%. However, MEP engineering studies have shown that when occupants have access to natural ventilation, the range of temperatures that feel comfortable are more closely related to the outdoor temperature.
San Diego has a fairly mild climate. Glumac’s initial studies showed that during occupied hours, the outside air temperature was acceptable for the indoor environment almost 2,000 hours of the year.
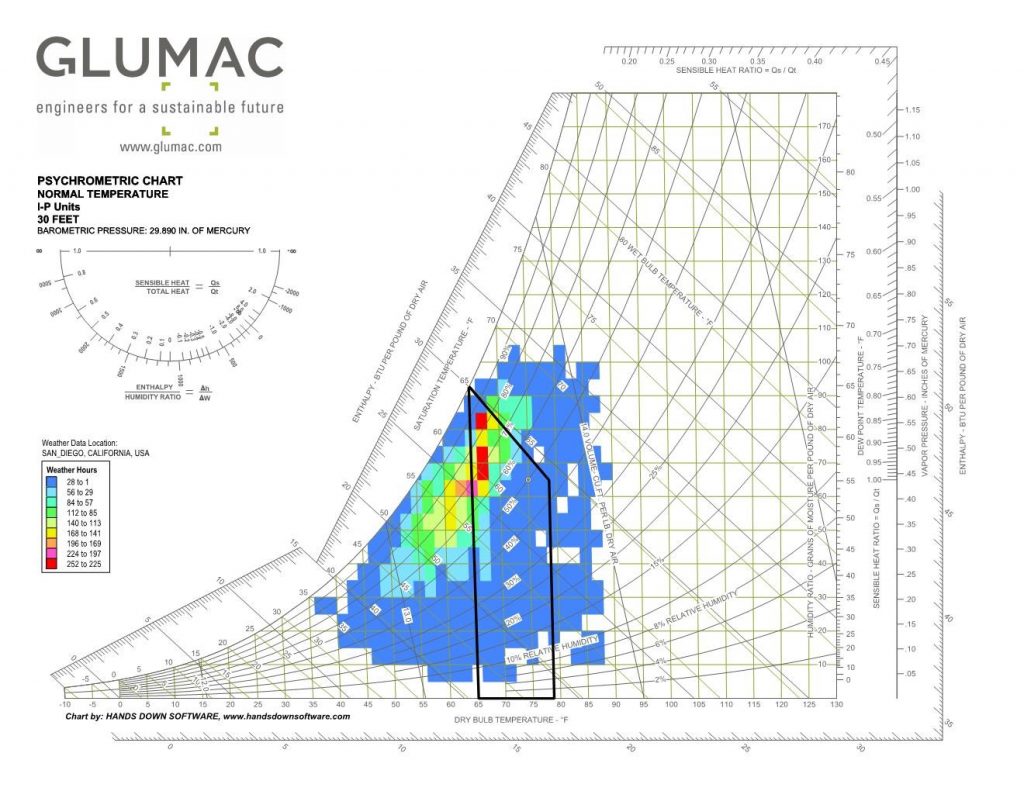
Glumac MEP engineering experts utilized CFD modeling to predict the indoor temperatures using natural ventilation, experimenting with different configurations of louvers, relief vents, and wind speeds. Because occasionally the outside air conditions may be too hot, too cold, or too humid for ideal office conditions, the team provided a mixed mode ventilation system. If the space was too hot or too cold, radiant finned tube radiators would provide supplemental heat, and chilled sails would provide supplemental cooling. During extremely hot, cold, or humid days, the natural ventilation system would close to switch over to 100% mechanical heating and cooling.
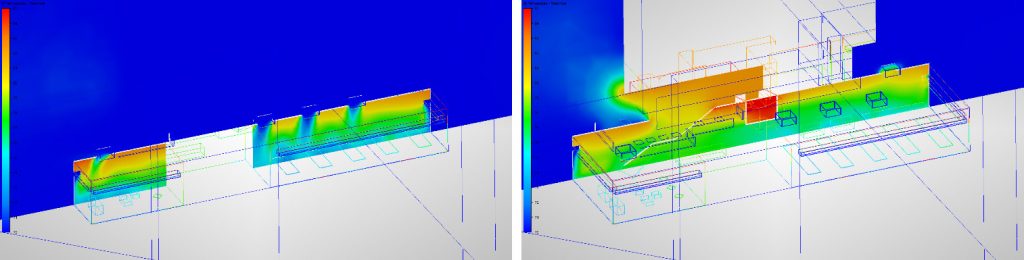
Healthy and Sustainable Building Design Integration
Fresh out door air is introduced into the space through an intake system. The intake system is composed of the following: an exterior louver and insect screen that prevent rain, insects, and airborne debris such as leaves, from entering the space; a control damper that allows the natural ventilation system to be closed during unoccupied periods or when the outdoor conditions are too extreme, allowing the space to be switched into full mechanical cooling or heating; the plenum is lined with a sound-absorbing material and creates a 90° elbow, which dampens the sound of the urban outdoor environment; a low pressure drop filter media that filters dust and mitigates some odors without drastically impacting the effectiveness of the natural ventilation system; and finally, a grille on the interior of the space that holds the filter media in place, and allows access into the plenum for service and maintenance.
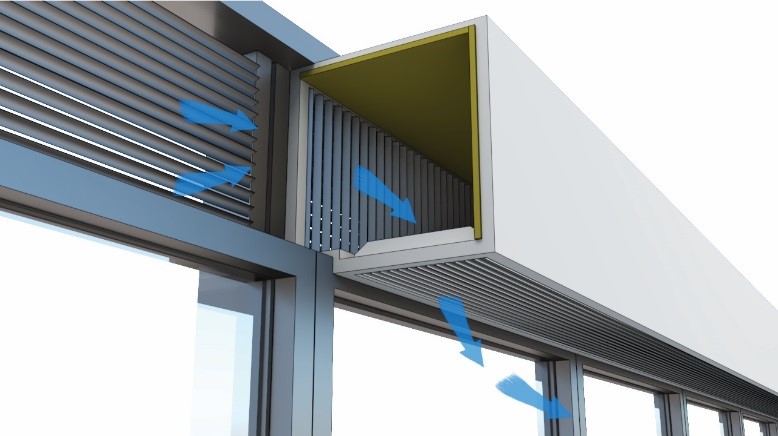
Finned tube radiators wrap around the exterior walls to handle heat losses from the exterior wall. The radiators work by hot water flowing through a continuous tube, which radiates heat through the metal fins. The radiation from the fins will warm the surrounding surfaces, such as the enclosure around them. The bottom of the enclosure is open, allowing cool air at the floor to enter the bottom of the enclosure. As the air warms around the fins, the hot air will rise up through the enclosure and out of the grille at the top. Control valves at each zone of radiators control the flow of water to adjust the temperature the radiator outputs. These control valves are automatically adjusted by the zone temperature sensor.
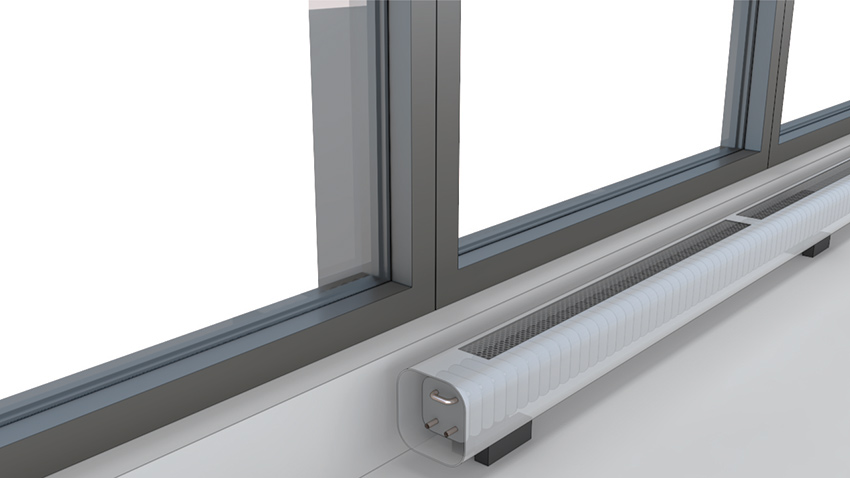
Chilled sail waves hanging throughout the space provide double, and in some cases, triple, the amount of cooling that can be provided through more standard chilled sails. Heat rises from the occupied space to the plane of chilled sails, through the slots in the sails and between them. Chilled water flows through tubes on the back side of each sail, cooling the surface of the sail. The wave profile provides extra surface area, and therefore, extra capacity. The hot air passes over and through the cool surface, which lowers its temperature. The cool air then drops back through the wave profile, inducing a current through the space, without the need of a fan.
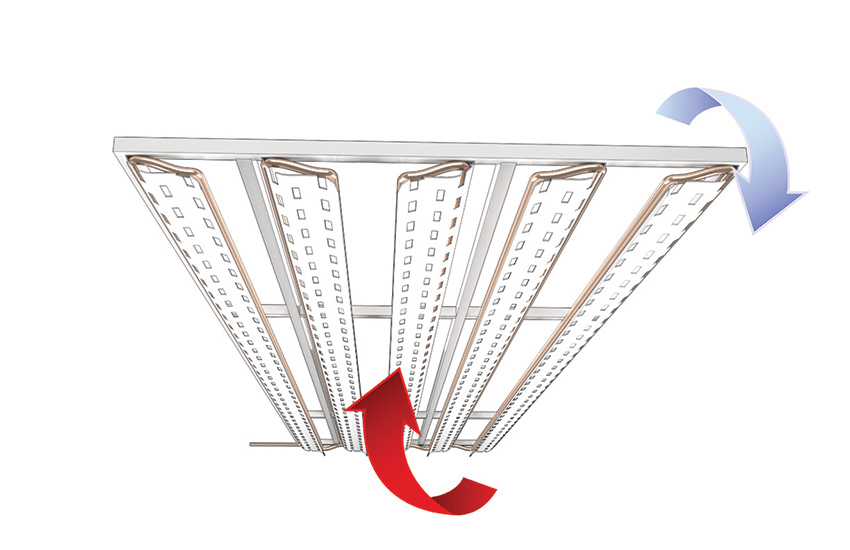
Chilled beams provide effective thermal comfort and energy savings for smaller spaces, such as the enclosed offices and mezzanine. Our MEP engineering experts integrated two different types of chilled beams, active and passive. The passive chilled beams operate similarly to the chilled sails described above. In passive beams, warm air rises without assistance into the beam. Chilled water flows through a coil inside the beam. As the warm air cools, the air drops back into the space, inducing a current. In active beams, fresh air is introduced into the supply air plenum. The plenum is pressurized, which forces air through nozzles inside the beam. The high velocity mixes room air into the air stream. The warm, mixed air, is induced through the chilled water coil, then cool air falls back into the space. A “Coanda” plate on the active chilled beam helps the fresh air mix with the room air in spaces without ceilings. The plate helps avoid the feeling of draftiness.
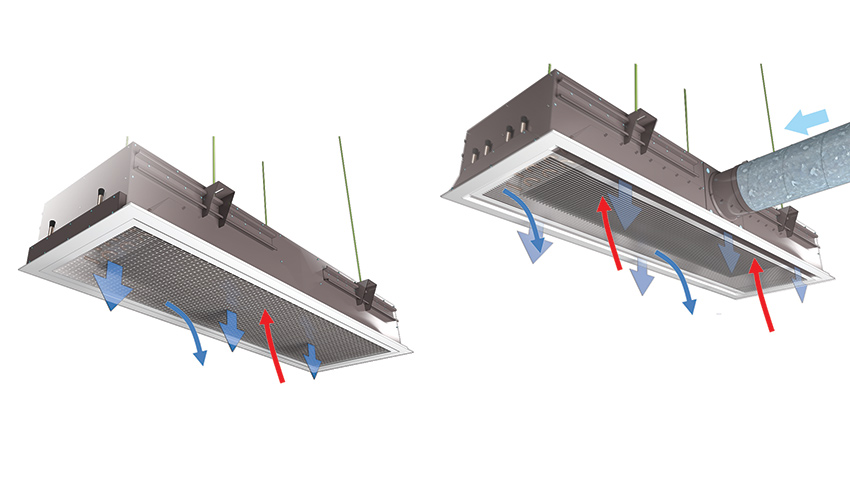
Lighting Design and Power
Expertly crafted architectural lighting is a crucial element to sustainable building design. The lighting and power systems were designed to be both energy-efficient and practical. The lighting system makes use of efficient LED fixtures and lighting controls while the power system utilizes the lighting system’s sensors to minimize energy usage.
Efficient LED luminaires were selected for all lighting in the space. We chose fixtures to meet the lighting needs of the occupants and the tasks while using the least amount of energy and developed a lighting design that puts light on the work planes and to highlight areas, but minimizes where it was not needed or desired. The ceiling and floor materials and colors were also selected to help the efficiency of the lighting. White ceilings and polished floors reflect more light, allowing more surfaces to be lit due to bounced indirect light, without adding additional light fixtures.
Designers selected light fixtures of either 3500K or 4000K – a combination that contributes to both aesthetics and efficiency. 4000K is more efficient and gives a higher perceived brightness with less wattage, while still being a comfortable color of light under which to work. It was specifically chosen in the most heavily daylight areas because it matches the color of daylight more closely than 3500K. In the mezzanine, we incorporated color-tunable lighting for areas where different architectural samples will be viewed and selected for projects. The color tuning allows users to adjust the color temperature of the lights warmer and cooler (from 2700K-6500K) to mimic the spaces of their projects. For example, they would want to view the samples under warmer light (2700K) for a residential or restaurant project and under cooler light (4000K) for a retail or healthcare project. The users could also view samples of exterior materials under 6500K to most closely mimic daylight. In the open offices on the first floor, indirect (up-light) fixtures were mounted high on columns to make the space seem larger, keep the ceiling plane clear, and avoid any visual glare.
Learn more about MEP engineering and sustainable building design experience at our project portfolio on glumac.com
The California Energy commission sets limits for the maximum wattage that can be installed in different spaces depending on the square footage and space type. By selecting fixtures that have high efficacy, the installed wattage of the lighting system reached a 36% reduction in the allowed lighting power for the project.
Lighting fixtures are controlled throughout the space by a system of lighting controls. Lights in the open offices, corridors, and main lobby are controlled by an astronomical timeclock in the electrical room so lights automatically turn on during business hours and defer to occupancy sensors after hours. The timeclock has 365-day programming that accounts for the length of day during different seasons and holidays. Dual-technology occupancy sensors with infrared and ultrasonic sensors detect the movement and sound of occupants and turn lights off after 30 minutes of vacancy. Photocells measure lighting levels on the work surface and dim lighting fixtures in daylit zones to conserve energy by harvesting the available daylight that enters the space. Lights in daylit zones are automatically raised/lowered to maintain minimum lighting levels on the surfaces below. Dimming wall stations allow manual input to further dim lights or temporarily override the controls sequence. Controlled receptacles reduce the power consumption of nonessential electrical devices left operating in unoccupied rooms. Receptacles installed throughout the office, lobby, conference rooms, kitchen areas, lounges, and copy rooms are interconnected with the occupancy sensor(s) in each room to automatically shutoff devices like monitors and portable lamps after 30 minutes of inactivity. Each controlled receptacle is permanently marked to differentiate them from uncontrolled receptacles, to avoid shutting off power to plug loads that should not be switched off.
We’re Proud to Announce the Newest Members of Our Leadership Team
They’re joining our leadership group because of their commitment to sustainability in the built environment, innovative building design, and most of all – our clients. These new leaders will continue to steer Glumac forward in delivering best-in-class projects. Feel free to reach out and discuss how we can collaboration something great: [email protected]. |
![]() |
DAVID NELSON, PE, LEED AP BD+C Senior Vice President | Los AngelesDavid leads Glumac Los Angeles’ Electrical Department, and is one of the leaders of our Healthcare group – focusing on the integration of innovative and energy-efficient design strategies in hospitals, laboratories, and medical office buildings. Over his career, David has developed a reputation for successfully directing engineering groups and coordinating efforts with other disciplines and stake holders to deliver successful projects – including major systems and infrastructure upgrades for the Kaiser Medical Center in Panorama City, CA and the LEED-certified renovation of Kaiser’s Santa Clarita, CA Medical Office Building. |
![]() |
NICOLE ISLE, WELL FACULTY, LEED AP BD+C, BADT
Vice President | Portland As Glumac’s Chief Sustainability Strategist, Nicole champions sustainable design strategies and is an authority on green building certifications – leading clients through innovative approaches to achieving LEED and WELL certifications in the healthcare, higher education, and commercial market sectors, most recently at Portland’s Hassalo on Eighth mixed-use EcoDistrict and Daimler Trucks North America Headquarters. She is also an intellectual steward, keeping Glumac’s thought leadership on the cutting edge. Most recently, Nicole presented at Greenbuild 2017 on linking science and design.
|
![]() |
KAMERON BEEKS, PE, LEED AP BD+C, WELL AP
Vice President | Los Angeles Kameron has become a leader in sustainable mechanical design, with an expertise in radiant systems, active chilled beams, vacuum plumbing technologies, and dashboarding for performance measurement and reporting. Kameron is an active green building thought leader – becoming a Living Building Challenge Ambassador and one of Glumac’s first WELL APs. Working on a diverse array of projects, Kameron has recently worked on the Port of Los Angeles AltaSea project, which is currently pursuing Net-Positive Energy, and the Wilshire Grand Tower in downtown Los Angeles.
|
![]() |
JEFF KLOMPUS, PE
Vice President, Director | San Francisco Jeff brings a career’s worth of energy-efficient consulting to Glumac, with a specialty in legislative policy and data analysis. Jeff has managed teams of engineers in solar PV feasibility analysis, procurement support, and construction. Jeff manages Glumac’s San Francisco office and firm-wide higher education group.
|
![]() |
SEBASTIAN ASVARAKSH, PE Vice President | San FranciscoSebastian has a wide array of design experience, with a focus on energy modeling, site optimization studies, life-cycle cost analysis, and renewable energy system modeling. He has worked on projects across four continents in the commercial and mission critical sectors, most notably completing mechanical designs for Google’s multi-floor San Francisco office, Oracle’s modular tier-3 data center development in Utah, and Samsung’s R&D facility in Mountain View, California. He is also one of Glumac’s Revit experts, providing user leadership and training. |
![]() |
QUINNIE LI, WELL FACULTY, LEED AP Vice President | Shanghai Quinnie’s work in helping develop the sustainable design industry in China has lead to incredible success for our Asia group. She has lead major commercial headquarters projects for clients including Alibaba and Jones Lang LaSalle, and provided energy analysis and sustainability benchmarking for Daimler’s Shanghai office, and China’s first Living Building Challenge registered project – our own headquarters in Shanghai. |
At this point, a lot of digital ink has been spent discussing the benefits of radiant heating and cooling systems in the built environment. Traditional forced-air systems, while cheaper to install, use more energy to properly condition a space. Radiant solutions often recommended by MEP engineering experts because they are more effective in providing and controlling occupant comfort than forced-air systems.
However, there remains a significant hesitation toward integrating radiant technology across building market sectors, due in large part to higher upfront costs of installation. For example, the addition of radiant slabs may add about $10/sq.ft. on average to up-front costs of a traditional mechanical system. However, there are several downline avenues for return on investment that offer owners the opportunity to recoup that cost.
“At $34.19/sq.ft., re-purposing the saved areas as usable leasing space instead of mechanical rooms added up to an additional $302,000 in annual non-energy benefits.”
A major benefit to radiant, which we’ve discovered in our own work, comes from the space saved by reduced equipment size. The use of radiant technologies drives down energy consumption of a building and reduces space taken up by the HVAC system by piping water through the building instead of ducting air. Radiant systems do not completely eliminate the need for ductwork in a building – ventilation is still required by code to bring fresh air into the building. Radiant systems are often coupled with dedicated outside air systems to provide code-required ventilation air to the building, and to decrease the incidence of “dead zones” due to stagnant air. However, the use of radiant technologies drastically decreases the amount of space needed for ductwork as well as associated up-front and installation costs for air-side material. Pipe risers take up less space than shafts at the building core, increasing valuable rentable square footage for building owners. Glumac’s MEP engineering team performed an analysis on the 500,000 sq.ft. Edith Green-Wendell Wyatt building modernization project in Portland, Oregon, and found that using radiant technology saved 8,833 sq.ft. in mechanical space. At $34.19/sq.ft., re-purposing the saved areas as usable leasing space instead of mechanical rooms added up to an additional $302,000 in annual nonenergy benefits. Over the lifetime of a building, this can add up to significant returns.
Radiant cooling and heating involves piping chilled water or hot water through a material that can transfer heat effectively in the space. This method is most commonly extended to chilled sails, in-floor radiant slab, radiant ceiling panels, and radiant walls. Piping water through these materials requires significantly less transport energy than moving air with a forced-air system. Additionally, the air-side of an HVAC system is decreased in a radiant application, thereby decreasing fan and duct sizes, further decreasing the energy usage of the system. This creates sustained and predictable savings on monthly energy bills.
“The radiant heating and cooling system we designed at City Creek Center in Salt Lake City, provides a measured annual energy savings of 24% compared to a typical retail center.”
For example, the radiant system Glumac’s MEP engineering team designed at City Creek Center in Salt Lake City, Utah, provides a measured annual energy savings of 24% compared to a typical retail center in the same climate zone. The main shopping concourse features radiant heating and cooling, designed to condition with natural ventilation, heat with passive solar heating through operable skylights, and provide forced air heating and cooling at the very extreme ambient temperature conditions. The site is in full radiant and natural ventilation mode for 84% of the year, utilizing the forced-air systems for full cooling only 6% and full heating 10% of the year. The radiant system enabled the owner to dramatically decrease the carbon footprint of the site while creating a comfortable shopping environment.
As we continue to push for sustainable building design approaches, the business case remains a crucial part of the conversation. The radiant approach provides various possibilities for return on investment for owners through energy savings and by increasing usable leasing square-footage, as well as reducing space taken up by the mechanical system. But what’s more, this approach comes with the added benefit of increases to occupant health and productivity – a subject we will touch on in part two of this article.
Energy efficiency continues to weave itself into the expectation of building design. Building trades, including Mechanical, Electrical, Plumbing, and Structural Engineers, are having to modify their general practices. The new requirements of building codes, namely energy codes, are subsequently forcing age-old structural detailing into a new realm. Moreover, as designers seek to get the most bang for their buck in their building designs, the integration of structural design with mechanical, electrical, and plumbing (MEP) infrastructure becomes paramount.
High-performance building design requires everyone be around the same table from day one, constantly evaluating the tradeoffs from one concept to another. This article discusses three of the most prominent relationships between structure and energy efficiency: structural type, application of rigid insulation, and the elimination or reduction of thermal bridging.
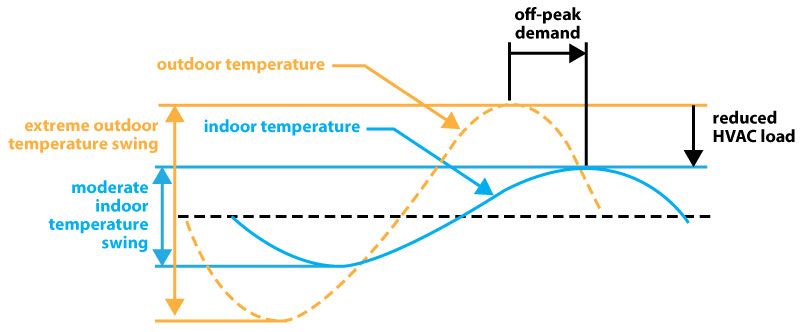
The first step in the design conversation involves the selection of structural materials. In most cases, this is a question of steel or concrete, although high-performance building designs are driving other solutions as well, such as straw-bale construction, structurally-insulated panels (SIPs), phase-change materials (PCMs), and polymer-based 3-D printing. Regardless of the structural material, the concerns associated with thermal massing, thermal breaks, and application of insulation remain the same. For example, a concrete structure has an inherently larger thermal mass capacity than steel-framed structures (because heat dissipates from steel faster than concrete). Thus, concrete can provide exceptional thermal comfort, reduced/delayed peak loads (Figure 1), and improved energy efficiency when applied properly. Of course, span length, seismic weight, commodity pricing, and other issues can override these concerns, which forces decisions regarding structural type. Design teams must consider their local climate to drive material selection. Milder climates, for example, tend to support low thermal mass, while more extreme climates tend to support (properly applied) high thermal mass.
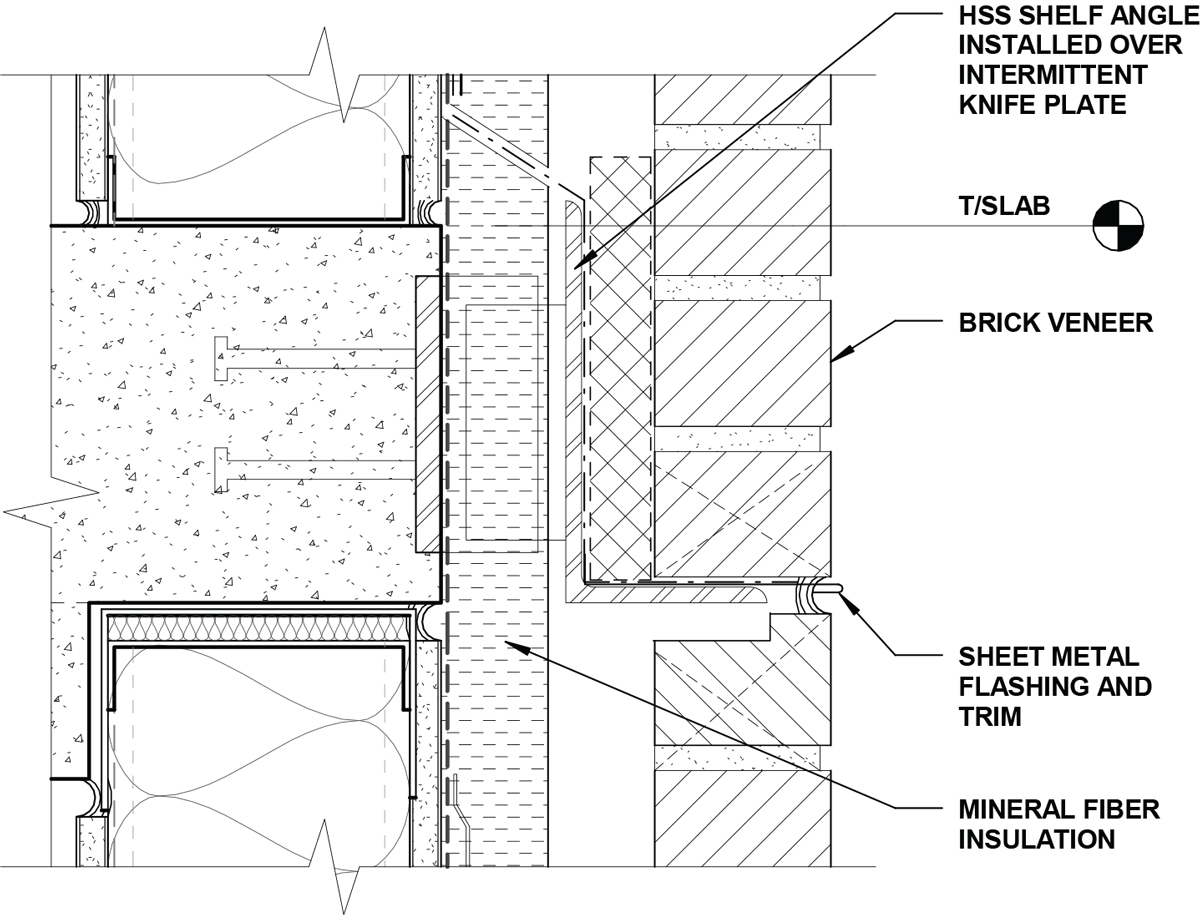
The default response is to add more insulation to drive down energy usage in buildings. The traditional application of fiberglass batt insulation becomes limiting because increased thermal resistance values (R-values) demand thicker walls. A point of diminishing return is reached quickly as usable floor area is decreased and wall thicknesses require significantly more structure to stand up. Theoretically, the “silver bullet” solution to this challenge is the application of rigid insulation. It has about twice as much R-value per inch as traditional batt insulation and can maintain a relatively similar thermal performance, regardless of the structure type, due to its theoretically “continuous” application that is not disrupted by structural members. On paper, this is easy to visualize, and the R-value numbers look great; however, the reality of its application in the field is not so straightforward. For example, the 2012 International Energy Code (IECC) expects a U-value of 0.079 Btu/hr-sf-°F as a prescriptive minimum for metal stud walls. With a 2×6 metal stud wall, this equates to approximately R-21 batt insulation between studs with 1.5 inches of rigid insulation applied to the exterior. Not only can this target U-value not be reasonably achieved in metal stud walls without the integration of rigid insulation, but the choice of exterior finish can have additional structural challenges. If plaster is applied as the exterior finish, then all is good. If brick is applied, then the face of the wall is upwards of 5 to 6 inches away from the supporting studs, with the heaviest part (brick) at the furthest point (Figure 2). This conundrum either forces the designers to dramatically increase the structural elements, such as shelf angles and the detailing associated with this assembly, or select different materials and assemblies altogether.
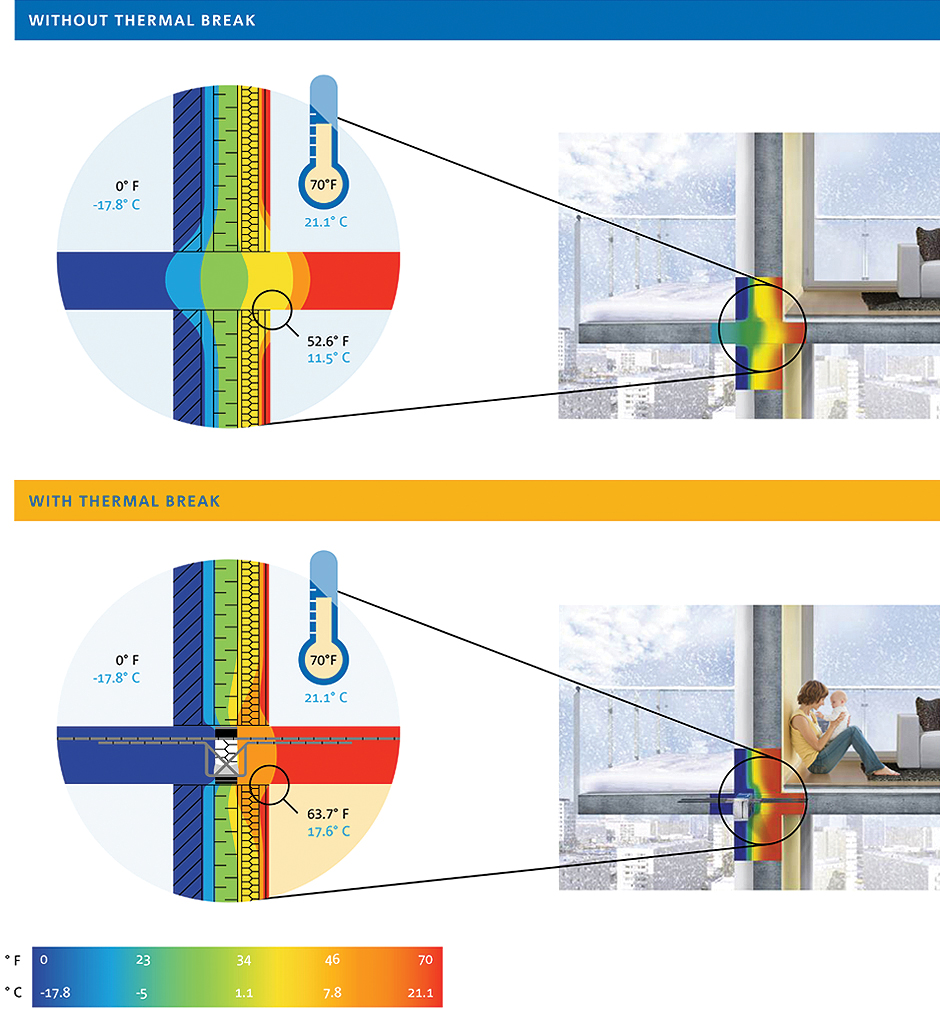
In addition to increased insulation values, appropriate attention to thermal bridging and the incorporation of thermal breaks are becoming important considerations. The objective is to eliminate or minimize all direct connections for heat transfer between the outside and the inside of the building structure. Although relatively small in area, the number of connection points is high across an entire building, which ultimately degrades the theoretical performance of the building envelope assembly in a big way. Imagine a metal screw or pin that holds the rigid insulation to the metal structure. This point is a direct pathway for the outside temperature to influence the inside temperature, which defeats the whole purpose of applying insulation in the first place. This phenomenon (Figure 3) is inherent in numerous envelope assemblies: metal-framed windows, rigid insulation applied to metal stud, and concrete structures where floors and walls meet (to name a few). The challenge with these thermal pathways is that they de-rate the U-value of the overall assembly, which requires the application of more (or higher performance) materials/systems to offset this deficiency. In metal stud framing without continuous insulation, the performance of the wall can degrade upwards of 50% from the prescribed insulation R-value due to thermal bridging of the studs passing through the batt insulation. For this reason, the 2016 California code now mandates (i.e. not allowed to circumvent with whole-building energy analysis) rigid insulation on metal-framed 2×6 stud walls. Furthermore, if not addressed appropriately, the impacts of thermal bridging can cause moisture problems within the assembly, which has a multitude of problems (e.g. mold, structural degradation, etc.). Infiltration can be avoided when a tightly sealed continuous insulation layer is applied to walls. To avoid the pitfalls associated with thermal bridging, structural engineers must envision how the envelope and structural assembly come together in a way that maximizes the application of continuous insulation.
This evolution in the building industry requires unprecedented integration and coordination during the design process. All parties need to be engaged early in the project, and decisions cannot be made in a vacuum. Energy modeling from concept through completion is necessary to realize the high-performance expectations of the industry and codes. By asking the questions early on and modeling the energy/cost performance of multiple scenarios, the chances of delivering the product that the client envisions (and codes expect) becomes much more feasible.